Translate this page into:
Molecular and qualitative characterization of compatibility between valacyclovir hydrochloride and excipients as raw materials for the development of solid oral dosage formulation
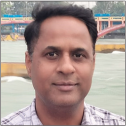
*Corresponding author: Ashok Kumar Yadav, University Institute of Pharmaceutical Sciences, Panjab University, Chandigarh, India. ashoky@pu.ac.in
-
Received: ,
Accepted: ,
How to cite this article: Mishra A, Sinha VR, Sharma S, Mathew AT, Kumar R, Yadav AK. Molecular and qualitative characterization of compatibility between valacyclovir hydrochloride and excipients as raw materials for the development of solid oral dosage formulation. Am J Biopharm Pharm Sci. 2023;3:8. doi: 10.25259/AJBPS_12_2023
Abstract
Objectives:
The objective of this present study is to know the compatibility of valacyclovir hydrochloride (VCH) with common excipients that would be utilized to develop solid oral dosage forms. Several spectroscopy techniques were used to know the possible interactions of VCH with excipients. More, a molecular docking study was also carried out to see the interaction of VCH with excipients. In vitro study of a physical mixture of VCH with excipients was executed to know the release of a drug.
Material and Methods:
Several analytical techniques such as differential scanning calorimetry, nuclear magnetic resonance spectrometer, and Fourier-transform infrared (FTIR) spectroscopy have been utilized to know the drug-excipient compatibility. Further, possible interactions between valacyclovir and different excipients were assessed by thin-layer chromatography. In vitro dissolution studies in different sets of experiments were done to determine the influence of the hydrophobic and hydrophilic nature of excipients (on the dissolution profile of VCH using USP II-type dissolving apparatus). Moreover, in silico molecular docking studies were also done to know any possible molecular interactions among drugs and excipients using AutoDock VINA 1.2.0 software and GROMACS 5.0 software.
Results:
FTIR and 1H NMR spectra of VCH and physical mixtures of VCH and excipients were compared and it was observed that no significant deviation of characteristic peaks in infrared spectroscopy and 1H NMR signals was detected. The endothermic peak of VCH in the physical mixtures of drugs and excipients was found in approximately the same position. In vitro dissolution studies displayed the influence of the hydrophobic and hydrophilic nature of excipients on the dissolution profile of VCH. For the physical mixture of VCH with lactose (LAC) and dicalcium phosphate (DP), % drug release was found to be 31.96% and 33.16% at 10 min, whereas the amount of % drug released for the mixture of VCH and talc was 25.00%. For two other excipients such as LAC and DP, the % drug release was determined to be 42.96% and 41.64%, respectively, for 30 min. The docking study also provided insights into the lowest energy conformations. Docking study anticipated that the number of interactions were more between valacyclovir and LAC (four nos.) in comparison to valacyclovir and microcrystalline cellulose (MCC) (two nos.). This interaction showed that in vitro drug release for the physical mixture of VCH with MCC was higher than a mixture of valacyclovir with LAC.
Conclusion:
A compatibility study of VCH by analytical techniques established that VCH was compatible with utilized excipients. Drug dissolution of VCH and physical mixture of MCC exhibited the maximum amount of drug release whereas a mixture of VCH with magnesium stearate released the minimum amount of drug for both short (10 min.) and long (30 min.) period. Docking studies disclosed that the LAC complex showed less deviation with less root mean square deviation value in comparison to the microcrystalline complex. Thus, the LAC complex has more hydrogen bonds and it was more stable as compared with the MCC complex. Therefore, VCH and used excipients could be used for solid dose formulations.
Keywords
Valacyclovir hydrochloride
Compatibility studies
Docking study
Excipients
In vitro dissolution
INTRODUCTION
Any new drug development process is built around four pillars of safety, efficacy, quality, and stability of a formulation. Understanding the physicochemical properties of both the active pharmaceutical ingredient (API) and all other components such as excipients, manufacturing aids, and packaging materials of the drug product is crucial if these qualities are to be consistently maintained in a finished dosage form.[1] During the preformulation stage for the development of a new drug, detailed studies of the API and other formulation ingredients are typically done.[2,3] Through the preformulation stage, the API is characterized in terms of its solubility, dissolution, permeability, polymorph/salt screening, stability (in the solid and solution phase), ionization properties, particle size distribution, API-excipient compatibilities, etc.[4] Every pharmaceutical formulation contains excipients, which aid in the production, stability, administration, distribution, and/or other capabilities of the dosage form.[5] Excipients are utilized to enhance product performance (e.g., modified drug release), improve processing (e.g., enhancing powder flow, and powder compatibility), and or facilitate patient compliance (e.g., flavor masking). There is a chance of physical and or chemical interactions between the API and one or more excipients in a formulation due to their proximity. The physical stability or performance characteristics of the drug product could be negatively impacted by any such interactions.[6] To prevent these side effects, to make it easier, and to create a solid and efficient formulation, the choice of excipient is of utmost importance. Thus, excipient-API compatibility screening is acknowledged as a key component of formulation development for a rational selection of excipients. In addition, the International Council on Harmonization Q8 guidelines and the USFDA’s 21st-century current good manufacturing practices initiative include expectations of a clear understanding of any interactions between the formulation components.[7] In addition, recent developments in several thermal and nonthermal analytical techniques have increased the effectiveness in the early detection, monitoring, and prevention of incompatibilities for the drug development process.[8] A scheme for the pre-formulation parameters to develop solid dosage forms is given below [Figure 1].
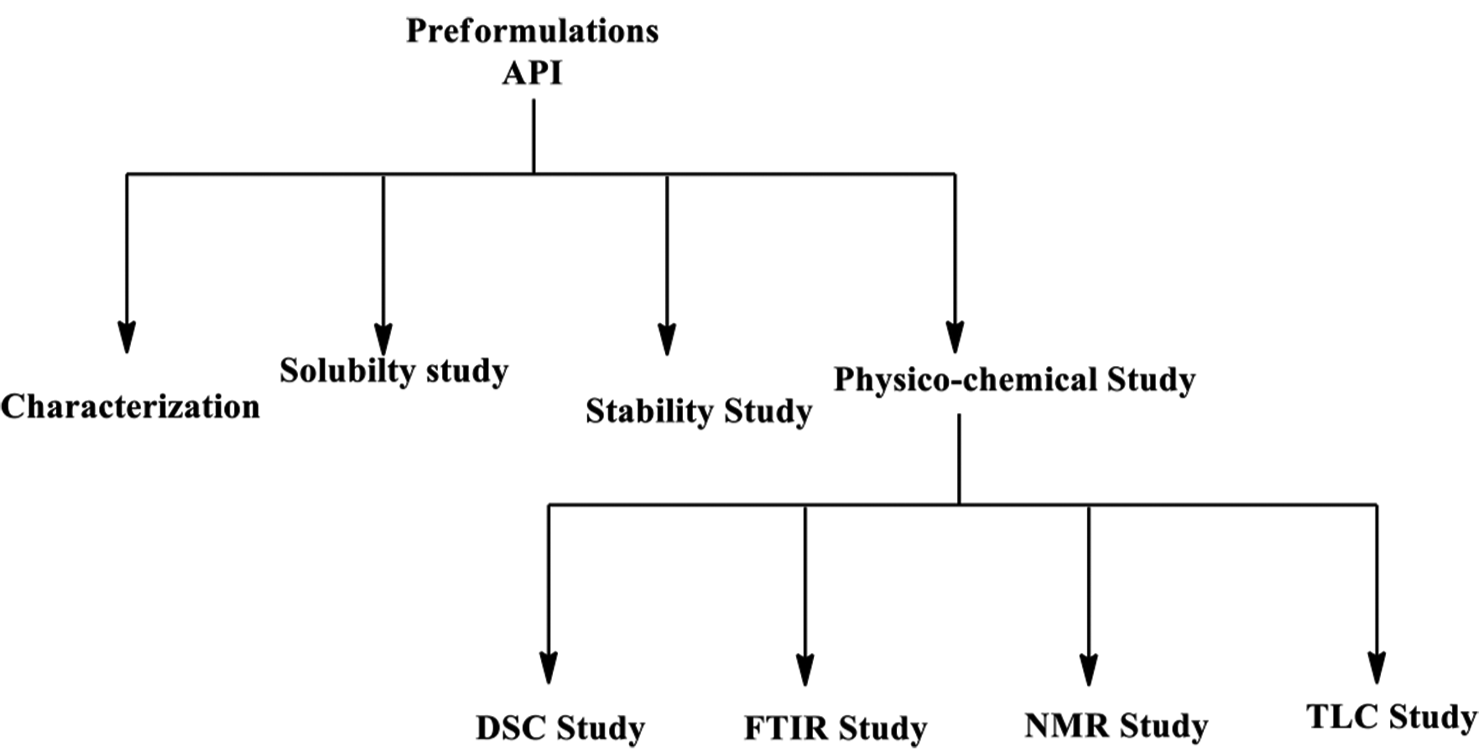
- A scheme for the pre-formulation parameters to develop solid dosage forms.
The antiviral drug valacyclovir hydrochloride (VCH) is primarily used to treat herpes simplex virus, cytomegalovirus, and other viral infections such as varicella-zoster virus and Epstein-Barr virus.[9] This drug has been chosen so that the impact of drug-excipient compatibility can be studied. The purpose of this study is to know any type of drug-excipient incompatibilities, as well as the most recent developments and methods for assessing them while formulating new drugs.[10] The compatibility study of VCH with excipients such lactose (LAC), magnesium stearate (MS), microcrystalline cellulose (MCC), talc (TALC), and dicalcium phosphate (DP) has not yet been studied till date. Utilizing cutting-edge analytical techniques, the present study assesses the drug-excipient compatibility testing of VCH. Nuclear magnetic radiation, differential scanning calorimetry (DSC), ultraviolet (UV) spectroscopy, and infrared spectroscopy are used to assess the interactions between the drug and excipient. Many kinds of drug-excipient interactions can be predicted with the use of these various analytical techniques. In addition, docking studies are conducted to determine how a protein interacts with a ligand. In this investigation, docking studies were utilized to identify potential interactions between the medication molecule and excipients. The docking study also sheds light on the conformations with the lowest energy.
MATERIAL AND METHODS
Materials
LAC, MS, TALC, and dicalcium phosphate were obtained from Loba Chemie Pvt. Ltd., Mumbai, and Central Drug House, New Delhi, respectively. VCH was managed by Dr. Reddy Pvt. Ltd., Hyderabad, India, as a gift sample for research purposes. From HiMedia Laboratories Ltd. in Mumbai, India, we bought MCC. Thermo-Fisher Scientific India Pvt. Ltd., Mumbai, India-based company, provided the solvents.
Methods
Compatibility studies
Physical mixing of VCH and excipient was done in a 1:1 ratio and a compatibility study of VCH with common excipients was conducted.
Fourier-transform infrared (FTIR) spectroscopy studies
Using FTIR spectroscopy, the various functional groups of the test substance are distinguished by their individual vibrational frequencies. FTIR can be useful for determining the compatibility of components since any change to a bond that exhibits distinct vibrational frequencies leads to spectral changes that produce frequency shifts and splitting in the absorption peaks. As a result, any spectrum alterations discovered during the compatibility study may indicate incompatibility. Using the KBr disk method with a sample-to-KBr weight ratio of 1:100, FTIR spectra were obtained in the range of 4000 cm−1–500 cm−1 using Spectrum RX 1 (Perkin Elmer, U.K.).[11,12]
DSC studies
DSC thermograms of the samples were acquired using DSC-Q20-TA instruments (New Castle, Delaware, U.S.). The process was followed exactly as it was outlined in the literature. Samples were heated in the nitrogen-purged sealed standard aluminum pans at a scanning rate of 10°C/min using an empty aluminum pan as the reference. DSC thermograms revealed interactions when new endothermic or exothermic peaks developed or disappeared, and component melting points significantly changed, or when changes in the reaction enthalpies were noted.[13,14]
Nuclear magnetic resonance (NMR) spectroscopy studies
The most used method for examining the structure of molecules is NMR spectroscopy. Due to the influence of nearby atoms on the signals from individual nuclei, NMR is a technology that is very sensitive to molecular structural characteristics. NMR spectrum of VCH was captured in DMSO-d6 using a Bruker Advance Neo 500 MHz NMR spectrometer.[15]
Thin-layer chromatography (TLC) studies
To separate the mixture of compounds, TLC is a technique which is used for analyzing mixtures. TLC is a sensitive technology that can analyze microgram (0.000001 g) amounts. A quick analysis can be completed in 5–10 min. Three steps make up TLC: Spotting, development, and visualization. To create an extremely diluted (approximately 1%) solution, the sample to be studied is first dissolved in a volatile (easily evaporated) solvent. Spotting is the process of applying a small amount of this diluted solution to one end of a TLC plate, in this instance a plastic sheet that has been covered with a thin layer of silica gel powder. The substance is only left in a small region after the spotting solvent and solvent have swiftly evaporated. The TLC plate is developed by submerging the bottom of the plate into a small pool of a separating solvent, which, then, rises on the plate due to capillary action. Methanol and triethylamine were utilized as the mobile phase in this study, with a ratio of 0.9:0.1. The solvent passes over the initial location as it advances up the plate. The separating solvent and the silica gel plate are put in competition for the spotted substance. Colored compounds are easily visualized. Direct observation of the spots is not possible after development. However, since most compounds lack color, a visualization technique is required. The TLC plate is infused with a fluorescent substance that lights when exposed to UV radiation. A spot that obstructs the fluorescence will be visible as a dark spot against a brilliant background. The spots can be traced with a pencil while they are exposed to UV light to identify their locations and get the Rf value.[16]
In vitro dissolution profile of VCH and its drug release mechanism
The dissolution investigations were carried out using a USP II-type dissolving apparatus. A beaker containing 900 mL of 0.1N HCl was used as the dissolution media, and 250 mg of VCH had been accurately weighed and placed into the capsule. The temperature was kept at 37 ± 0.5°C, and 100 RPMs were used for stirring. Samples of 5 mL were taken from each beaker at various times and replaced with new media. The same process was used to create a physical mixture of VCH and excipients. The samples that had been withheld were run through a 0.2 2 µm nylon filter and put through analysis at 252 nm using a Jenway 6850 UV/Visible Spectrophotometer. Accordingly, the release data were assessed for drug percentage dissolved in various excipients.
Molecular docking
The AutoDock VINA 1.2.0 software was used for docking operations. 2D structures of the compounds were retrieved from the PubChem database, and the Gaussian software was used to do preliminary geometric optimization at the DFT/B3LYP level of theory with the 6-311+G+ basis set. Using the AutoDock tools, the docking structures were constructed, and a blind docking with a grid size of 40*40*40 was carried out.[17-19]
Molecular dynamics (MD) simulations
The ideal docking stance produced by AutoDock VINA was used as the starting point for MD simulations. Using the GROMACS 5.0 program and a CHARMM36m force field, the VCH complexed with MCC and LAC was stimulated. Each of the stimulations used the TIP3 water model, and equilibration took place at 303 K. The GROMACS software suite was used to analyze the various interactions and trajectories.[20-23]
RESULTS
FTIR spectroscopy
Using KBr pellet technique, the FTIR spectrum of pure VCH was recorded on Spectrum RX 1, (Perkin Elmer, U.K). There was a scanning range 4000–400 cm−1. The FTIR spectrum of VCH is shown in [Figure 2 and Table 1], which is characterized by the absorption peaks at 3407.14 cm−1 (N-H stretch), 2923.08 cm−1 (C-H stretch), and 1734.63 cm−1 (C=O ester).[11,12]

- Fourier-transform infrared spectrum of (a) Valacyclovir hydrochloride (VCH), (b) physical mixture of VCH and lactose, (c) physical mixture of VCH and magnesium stearate, (d) physical mixture of VCH and microcrystalline cellulose, (e) physical mixture of VCH and talc, and (f) physical mixture of VCH and dicalcium phosphate.
Peaks | VCH | VCH+LAC | VCH+MS | VCH+MCC | VCH+TALC | VCH+DP |
---|---|---|---|---|---|---|
N-H | 3407.14 | 3384.42 | 3422.52 | 3402.60 | 3406.97 | 3409.37 |
C-H | 2923.08 | 2900.60 | 2920.24 | 2912.20 | 2920.52 | 2918.94 |
C=O ester | 1734.63 | 1733.43 | 1735.36 | 1731.20 | 1734.64 | 1734.96 |
FTIR: Fourier transform infrared, VCH: Valacyclovir hydrochloride, LAC: Lactose, MS: Magnesium stearate, MCC: Microcrystalline cellulose, DP: Dicalcium phosphate
1H NMR spectroscopy
1H NMR is the technique used to identify hydrogen content and its positioning. 1H NMR was performed on Bruker Advance Neo 500 NMR Spectrometer. A small amount of sample of valaciclovir hydrochloride was dissolved with DMSO-d6 in a narrow glass vial and positioned in the sample holder of the instrument. The 1H NMR of VCH exhibited peaks with characteristic values of chemical shift (δ) such as 0.91 δ (P and Q), 4.38 δ (G and J), 5.41 δ (E and F), 6.75 δ (D), 8.47 δ (B), and 10.81 δ (A) [Figure 3].[15]

- Structure of valacyclovir hydrochloride.
DSC
A small quantity of VCH (2.0 mg) was added and enclosed in the DSC aluminum pan. In addition, it was heated from 20°C to 250°C under nitrogen flow at a scanning rate of 10°C/min. As a reference, an empty aluminum pan was used. Endothermic peaks were noted. At temperatures 63.03°C, 81.60°C, and 128.16°C, the thermogram displayed three distinct endothermic peaks [Figure 4a-d] which were found to be like the reported thermogram of VCH.[13,14]
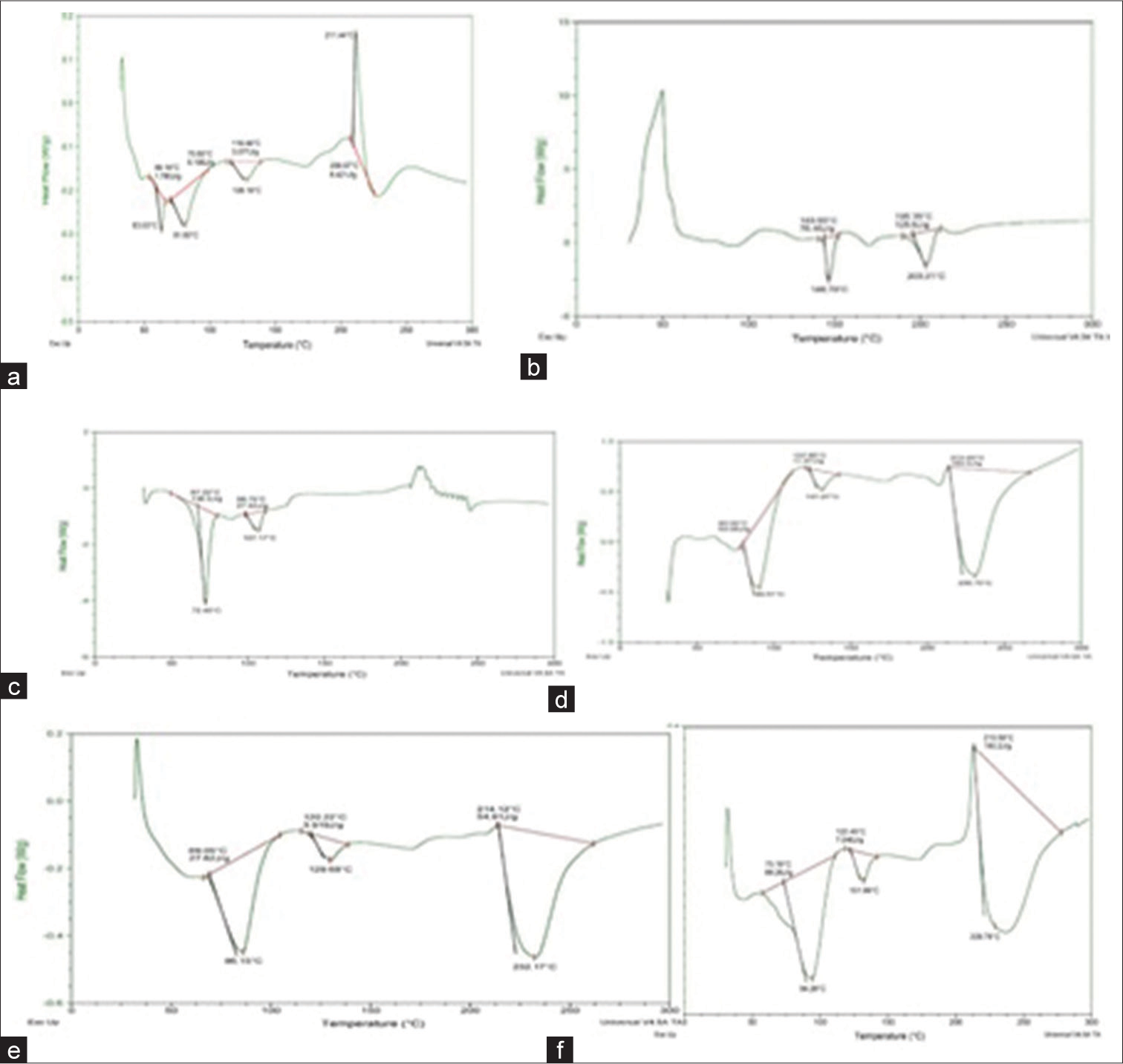
- Differential scanning calorimetry thermogram of (a) pure valacyclovir hydrochloride (VCH), (b) physical mixture of VCH and lactose, (c) physical mixture of VCH and magnesium stearate, (d) physical mixture of VCH and microcrystalline cellulose, (e) physical mixture of VCH and talc, and (f) physical mixture containing VCH and dicalcium phosphate.
TLC
The Rf value is used to calculate how far the materials moved along the plate. Rf is calculated by dividing the total distance covered by the material and the solvent. Its value is always a number between 0 and 1. VCH and physical mixes containing TALC, LAC, MS, MCC, and DP were shown to have a Rf value of 0.56 in triethylamine: methanol (0.1:0.9).
Influence of hydrophobic and hydrophilic attribute of excipient over dissolution profile
The dissolution behavior of VCH from the physical mixtures containing different types of excipients is shown in [Figure 5]. The % drug release of VCH varies depending on the excipient. The amount of VCH released should grow as time passes. For two excipients such as LAC and DP, % drug release was found to be 31.96% and 33.16% at 10 min, respectively, and the amount of drug release at time 10 min for other different excipients was found to be quite close. At time 10 min, it was observed that the amount of % drug released for VCH and mixture of VCH and TALC are 25.04% and 25.00%, respectively. Two other excipients such as LAC and DP displayed the % drug release at 42.96% and 41.64%, respectively, for 30 min. The physical mixture of VCH and MS was found to release 10% of the medication at 30 min [Figure 5].

- Dissolution profile of valacyclovir hydrochloride in different excipients.
Molecular docking
Typically, docking experiments were conducted to determine how a protein interacts with a ligand. In this investigation, docking studies were conducted to discover potential interactions between the excipients and the drug molecules. The docking experiment also sheds light on the conformations with the lowest energy.[17] The lowest energy conformer from each of the two tests was chosen as the best appropriate conformer in two docking investigations, VCH/MCC and VCH/LAC. The docking score for each complex was listed in [Table 2], and [Figure 6a and b] displays the 3D poses of the docked complexes VCH/MCC and VCH/LAC.
S. No. | Complex name | Docking score |
---|---|---|
1 | VCH/LAC | −2.6 |
2 | VCH/MCC | −2.6 |
VCH: Valacyclovir hydrochloride, LAC: Lactose, MCC: Microcrystalline cellulose

- (a) 3D docked pose of valacyclovir hydrochloride (VCH)/lactose and (b) VCH/microcrystalline cellulose.
MD simulations
The 10 ns simulations of valacyclovir in complex with MCC and LAC were examined for several energies and characteristics, including root mean square deviation (RMSD), root mean square fluctuation (RMSF), radius of gyration, and solvent assessable surface area.
The VCH/LAC complex is shown to have higher stability and fewer deviations due to its reduced RMSD, as shown in [Figure 7]. With the medicine, both excipients immediately establish equilibrium.

- Root mean square deviation (RMSD) plots for valacyclovir hydrochloride (VCH)/microcrystalline cellulose (red) and VCH/lactose (black).
The quantity of hydrogen bonds between the medication and excipient that were created throughout time is shown in [Figure 8]. The VCH/LAC complex forms more H-bonds than the VCH/MCC complex. Both complexes produce H-bonds, but they are not stable throughout the stimulus. For VCH/LAC and VCH/MCC, respectively, the average number of H-bonds produced during the stimulation per frame was 0.059 and 0.010.
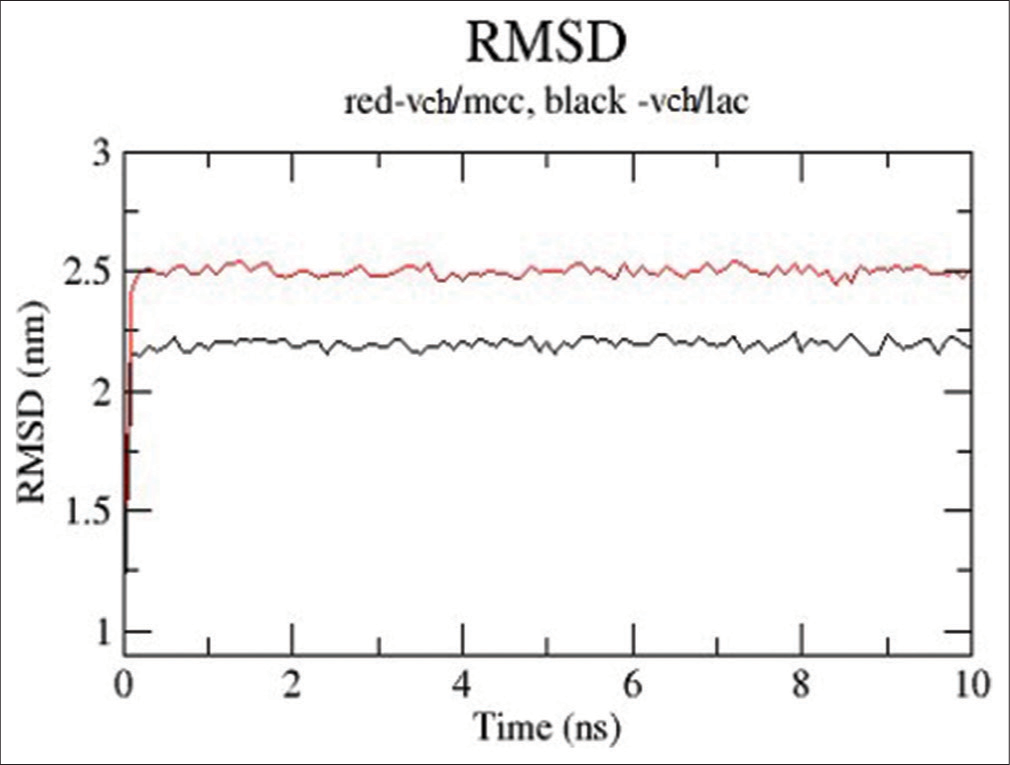
- Hydrogen bonds in each frame for valacyclovir hydrochloride (VCH)/lactose (black) and VCH/microcrystalline cellulose (red).
The compactness of the structure is determined by the radius of gyration. [Figure 9] demonstrates that the radius of gyration of the complex is not particularly stable and varies throughout stimulation. Comparatively speaking, VCH/LAC fluctuates less than VCH/MCC. The part of the ligand that is in touch with the solvent, in this case water, is known as the solvent-accessible surface. In addition, it gauges how hydrophilic the molecules. [Figure 9] shows that during most of the stimulation, the SASA of VCH/MCC is higher than VCH/LAC. This suggests that when VCH is complexed with MCC rather than LAC, it interacts with the solvent more.
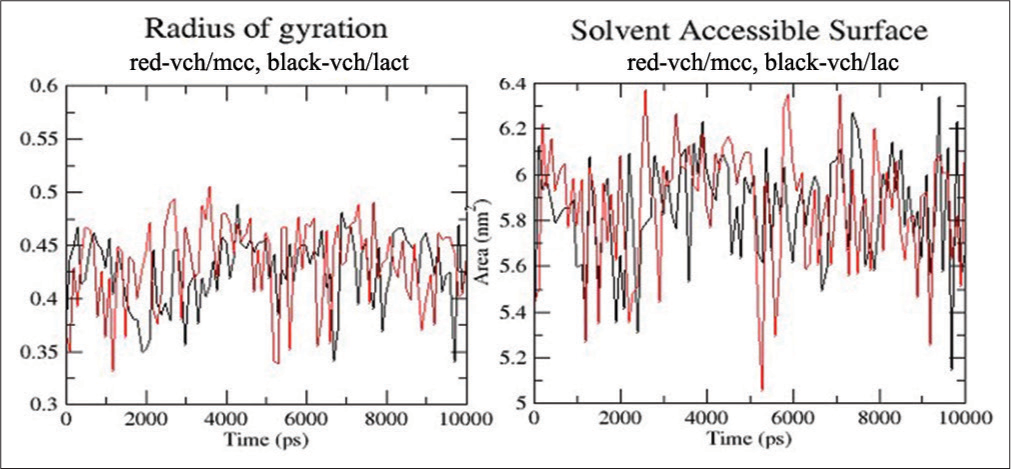
- Radius of gyration and solvent accessible surface area for valacyclovir hydrochloride (VCH)/microcrystalline cellulose (red) and VCH/lactose (black).
Along with total energy, the Lennard-Jones (LJ) and coulomb interaction energies of the medicine and excipient were determined and displayed. [Figure 10] clearly shows that the LJ interactions for both complexes were identical, while the coulombic interactions underwent a significant alteration. This suggests that the total energy is determined by coulombic interaction. The fact that the total energy of VCH/MCC (−43.4 Kj) is lower than that of VCH/LAC (−29.4 KJ) shows a stronger pharmacological interaction with MCC than LAC. This suggests that the medication is more soluble in the presence of MCC. The results of molecular docking support the dissolving findings, which showed that MCC released the most medicine when compared to LAC. This is because there is less H-bonding in MCC than LAC, as shown in [Figure 6a and b].
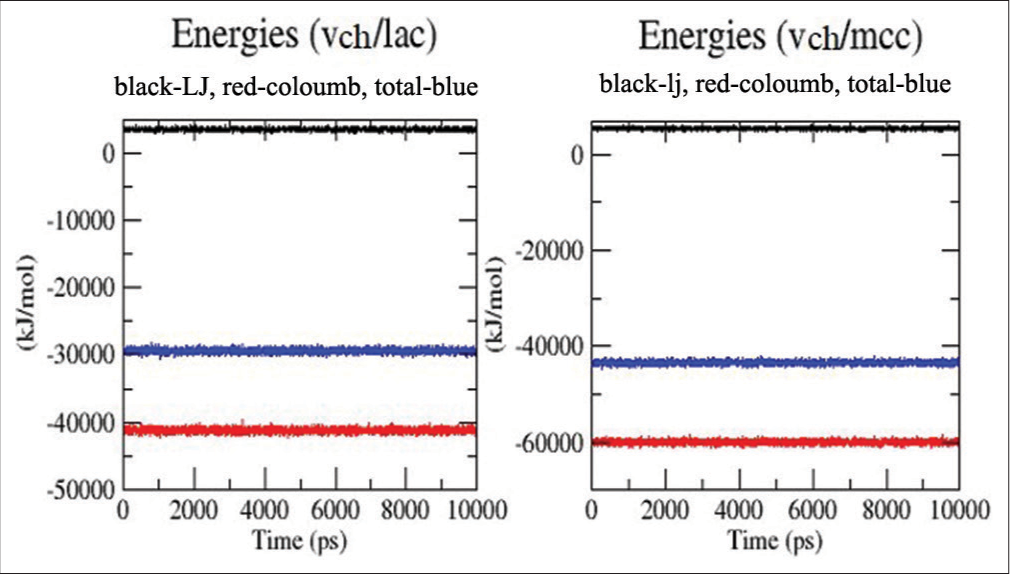
- Energy plots (Lennard Jones, coulombic and total) for valacyclovir hydrochloride (VCH)/lactose and VCH/microcrystalline cellulose.
DISCUSSION
FTIR studies
Identification of any possible incompatibilities between the drug and excipients is a major task to be achieved through compatibility studies. Compatibility studies deal with understanding any physicochemical interactions between drugs and excipients. Development of a robust and effective formulation necessitates careful selection of the excipients that maintain the quality, safety, efficacy, and stability of the drug product. FTIR is also a widely used technique to evaluate any incompatibilities between the drug and excipients. The FTIR spectroscopic analysis of the pure drug and physical mixture of excipient measures changes in the frequency and bandwidth of interacting groups during any physicochemical interactions. The FTIR spectrum of pure VCH is shown in [Figure 2a and Table 1], which is characterized by the absorption peaks at 3407.14 cm−1 (N-H stretch), 2923.08 cm−1 (C-H stretch), and 1734.63 cm−1 (C=O ester stretch). The absorption spectrum of the physical mixture of VCH and LAC is shown in [Figure 2b], which exhibited sharp peaks at 3384.42 cm−1 (N-H stretch), 2900.60 cm−1 (C-H stretch), and 1733.43 cm−1 (C=O) stretch). All the characteristic peaks corresponding to the drug and LAC were found to be present in a physical mixture. Moreover, there was no significant shifting of characteristic peaks or appearance of new bands which indicated that there was no significant interaction between the drug and excipient.
The absorption spectrum for the physical mixture of VCH and MS is shown in [Figure 2c], which showed sharp peaks at 3422.52 cm−1 (N-H stretch), 2920.24 cm−1 (C-H stretch), and 1735.36 cm-1 (C=O stretch). The characteristic peaks of the drug and MS were found to be present in the physical mixture nearly at the same position. It was also observed that there was no significant shifting of characteristic peaks or appearance of new bands.
The absorption spectrum for the physical mixture of VCH and MCC is shown in [Figure 2d], which displayed sharp peaks at 3402.60 cm−1 (N-H stretch), 2914.96 cm−1 (C-H stretch), and 1731.20 cm−1 (C=O stretch). All the characteristic peaks corresponding to the drug and excipient were observed in the physical mixture. No significant shifting of characteristic peaks or appearance of new bands was found in the spectrum of the physical mixture of drug and MCC which indicated no significant interaction between the drug and excipient.
The absorption spectrum of TALC is shown in [Figure 2e], which exhibited sharp peaks at 3406.97 cm−1 (N-H stretch), 2920.52 cm−1 (C-H stretch), and 1734.64 cm−1 (C=O stretch). All the characteristic peaks corresponding to the drug and excipient were found to be present in a physical mixture. Moreover, there was no significant shifting of characteristic peaks or appearance of new bands which showed no significant interaction between the drug and excipient.
The characteristic peaks for the physical mixture of VCH and DP are shown in [Figure 2f] and peaks appeared at 3409.37 cm−1 (N-H stretch), 2918.94 cm−1, (C-H stretch), and 1734.96 cm−1 (C=O stretch). The characteristic peaks corresponding to the drug and excipient were observed to be present in a physical mixture containing DP and VCH. This suggested that there was no interaction between the drug and the excipient.
1H NMR Studies
Most of the important signals of VCH showed peaks with characteristic chemical shifts at 0.91 δ (P and Q), 4.38 δ (G and J), 5.41 δ (E and F), 6.75 δ (D), 8.47 δ (B), and 10.81 δ (A) [Figure 3 and Table 3]. All the chemical shift corresponding to the physical mixture of drug and excipient were found to be present nearly at the same position. No significant shifting of chemical shift was observed in the physical mixture of the drug with the excipient which showed that no significant interaction between the drug and excipient occurred. Thus, it was concluded that VCH is compatible with all used excipients.
Chemical shift (ppm) | VCH | VCH+LAC | VCH+MS | VCH+MCC | VCH+TALC | VCH+DP |
---|---|---|---|---|---|---|
P and Q | 0.91 | 0.91 | 0.87 | 0.91 | 0.91 | 0.91 |
G and J | 4.38 | 4.40 | 4.33 | 4.39 | 4.40 | 4.39 |
E and F | 5.41 | 5.40 | 5.36 | 5.40 | 5.40 | 5.40 |
D | 6.75 | 6.70 | 6.68 | 6.72 | 6.76 | 6.76 |
C | 7.88 | 7.83 | 7.81 | 7.82 | 7.83 | 7.83 |
B | 8.47 δ | 8.59 | 8.59 | 8.59 | 8.59 | 8.59 |
A | 10.81 | 10.85 | 10.82 | 10.86 | 10.90 | 10.92 |
VCH: Valacyclovir hydrochloride, LAC: Lactose, MS: Magnesium stearate, MCC: Microcrystalline cellulose, DP: Dicalcium phosphate
DSC studies
The DSC thermogram of pure VCH reflected three sharp endothermic peaks at 63.03°C, 81.60°C, and 128.16°C [Figure 4a]. The DSC thermogram for the physical mixture of VCH with LAC was studied. [Figure 4b] depicted the DSC thermograms of the physical mixture of VCH and LAC. In the thermogram of the physical mixture of VCH and LAC, the endothermic peak corresponding to VCH was observed at 146.79°C. It was observed that the peaks corresponding to pure VCH were not retained, but when it was masked through TLC, it was observed that the spot of VCH and physical mixture of LAC with VCH was found to be at the same position. Through this, it was concluded that there was no chemical interaction between VCH and LAC which showed that it was compatible with LAC.
Further, DSC thermogram for the physical mixture of VCH with MS was studied. [Figure 4c] depicted the DSC thermograms of the physical mixture of VCH and MS. The DSC thermogram of pure VCH reflected three sharp endothermic peaks at 63.03°C, 81.60°C, and 128.16°C. In the thermogram of the physical mixture of VCH and MS, the endothermic peak corresponding to VCH was observed at 107.17°C. It was detected that the peak corresponding to pure VCH was not retained and when it was masked through TLC, the spot of VCH and physical mixture of MS with VCH was found to be at the same position. Through this, it was concluded that no chemical interaction between VCH and MS occurred. This showed that MS was compatible with VCH.
Next, the DSC thermogram of the physical mixture of VCH with MCC is shown in [Figure 4d]. The DSC thermogram of pure VCH showed three sharp endothermic peaks at 63.03°C, 81.60°C, and 128.16°C. In the thermogram of the physical mixture of VCH with MCC, the endothermic peak corresponding to VCH was observed at 90.51°C and 131.27°C. It is observed that the peaks corresponding to pure VCH were retained with slight modifications. When it was masked through TLC, it was observed that the spot of VCH and physical mixture of MCC with VCH were found to be at the same position. Thus, it was concluded that no chemical interaction between VCH and excipient. Therefore, MS was compatible with VCH.
Furthermore, the DSC thermogram of the physical mixture of VCH with TALC was studied [Figure 4e]. The DSC thermogram of the physical mixture of VCH and TALC displayed three sharps endothermic peaks at 63.03°C, 81.60°C, and 128.16°C. In the thermogram of the physical mixture of VCH with TALC, the endothermic peak corresponding to VCH was observed at 86.15°C and 129.68°C. It was observed that the peaks corresponding to pure VCH were retained with slight modifications and when it was masked through TLC, it was observed that the spot of VCH and its physical mixture with TALC was found to be at the same position. Through this, it was concluded that no chemical interaction between VCH and TALC was observed.
At the last, the DSC thermogram of the physical mixture of VCH (VCH) with DP is shown in [Figure 4f]. The DSC thermogram of the physical mixture of VCH and DP showed three sharp endothermic peaks at 63.03°C, 81.60°C, and 128.16°C. In the thermogram of the physical mixture of VCH with DP, the endothermic peak corresponding to VCH was observed at 94.28°C and 131.99°C. The peaks corresponding to pure VCH were retained with slight modifications. When it was masked through TLC, it was observed that the spot of VCH and the physical mixture of DP with VCH was found to be at the same position. Thus, it was concluded that there was no chemical interaction between VCH and MS which showed that it was compatible with VCH.
TLC
The Rf value is used to quantify the movement of the material along the plate. Rf is equal to the distance traveled by the substance divided by the distance traveled by the solvent. Its value is always between zero and one. The Rf value of VCH and physical mixtures with different excipients, that is, LAC, MS, MCC, TALC, and DP were found to be 0.56. The spot of VCH and all physical mixtures with different excipients were visible at the same position. From this, it was concluded that no interaction would take place between the VCH and all physical mixtures with different excipients.
In vitro drug release study
The dissolution behavior of VCH from the physical mixtures containing different types of excipients is illustrated in [Figure 5]. The VCH drug release is different for different excipients. As the time increases, the drug release should also increase. The amount of drug release at 10 min in different excipients was found to be quite near for two types of excipients, that is, LAC and DP which were found to be 31.96% and 33.16%. At 10 min, the amount of drug released for VCH and the mixture of VCH with TALC were found to be quite similar to that of pure drug, that is, 25.04% and 25.00%, respectively. The amount of drug released in the physical mixture containing MS with VCH was found to be 4.16%. The amount of drug release at 30 min in different excipients was found to be quite near for two types of excipients, that is, LAC and DP which were found to be 42.12.96% and 41.64%. The amount of drug released in the physical mixture containing VCH and MS was obtained at 10% at 30 min. Physical mixtures of VCH and MCC showed max. amount of drug release in a shorter period. On the other aspect, the physical mixture of VCH and MS showed a minimum amount of drug release in a longer period. The release of drugs from the physical mixtures containing different excipients was influenced by the dual effects of hydrophobicity and hydrophilicity of the different excipients.
Molecular docking
Docking studies were carried out usually to find out the interaction between protein and ligand. In this study, we used docking studies to find the possible interactions of excipients with the drug molecules. The docking study also gave insights into the lowest energy conformations. Two docking studies were carried out, that is, VCH/MCC and VCH/LAC, the lowest energy conformer of each of the two experiments was taken as the most suitable conformer. The docking score of each complex is shown in [Table 2] and the 3D pose of docked complexes VCH/MCC and VCH/LAC is shown in [Figure 6a and b].
MD simulations
The 10 ns simulations of valaciclovir in a complex of mcc and LAC was analyzed for different parameters such as RMSD, RMSF, radius of gyration, solvent assessable surface area, and various energies.
The RMSD plot given in [Figure 7] indicated that the VCH/LAC complex was having better stability and low deviations as its having lower RMSD. Both the excipients reached equilibrium very quickly with the drug.
[Figure 8] indicates the number of hydrogen bonds formed between the drug and excipient with respect to time. It is evident that VCH/LAC complex forms a greater number of H-bonds than VCH/MCC. The H-bonds formed by both complexes are not stable throughout the stimulation. The average number of H-bonds formed throughout the stimulation per frame was 0.059 for VCH/LAC and 0.010 for VCH/MCC.
The radius of gyration is a measure of the compactness of the structure. From [Figure 9], we can see that the radius of gyration of both the complexes was not very stable and fluctuated throughout the stimulation. VCH/LAC was comparatively less fluctuating than VCH/MCC. The solvent accessible surface was the area of the ligand which was in contact with the solvent, in this case, water. It was also a measure of the hydrophilicity of the molecules. From [Figure 9], we can see that for the majority part of the stimulation, the SASA of VCH/MCC was higher than VCH/LAC. This indicated that valaciclovir was more interactive with solvent when complexed with MCC than LAC.
The interaction energy of the drug and excipient in terms of LJ and coulomb interactions was calculated and plotted along with total energy. From [Figure 10], it was evident that the LJ interactions for both complexes were similar, and the change was drastic in terms of the coulombic interactions. This indicated that coulombic interaction was determining the total energy. The total energy of VCH/MCC (−43.4 KJ) was less than VCH/LAC (−29.4 KJ), indicating a strong interaction of the drug with MCC than LAC. This indicates that the drug was more soluble when combined with MCC. Molecular docking results also confirmed the dissolution results in which the maximum drug release was seen in the MCC when compared with LAC. This was due to less H2 bonding present in the MCC in comparison to LAC.
CONCLUSION
The compatibility studies of the drug (VCH) were studied by infrared spectroscopy and NMR. Further, it was observed that there was no change or minor deviation of the characteristic peaks in the infrared spectroscopy and NMR signals. Based on the above discussion, it was concluded that the drug VCH should be compatible with all types of excipients used in this study. Further to see the compatibility of the drug (VCH), DSC was done for VCH and its physical mixtures. VCH was found to be compatible with all types of excipients from DSC since there were no changes or slight changes in the observed peak of VCH in the physical mixtures. Further, some possible incompatibilities were ruled out through masked TLC. All the spots in the TLC of both the drug and the physical mixtures containing the drug and excipients came out to be at the same position which showed that there was no observed chemical interaction between VCH and different excipients. Thus, it was concluded that the drug should be compatible with all types of excipients. The drug dissolution behavior of VCH was also carried out. Physical mixture of VCH, and MCC showed max. amount of drug release in a shorter period. The release of drugs from the physical mixtures containing different excipients was influenced by the dual effects of hydrophobicity and hydrophilicity of the different excipients. Docking studies were carried out to find the possible interaction of drugs with excipients. The complex of VCH and LAC showed less deviation with less RMSD value as compared with MCC complex. LAC complex having more H2 bonding and is more stable as compared with the MCC complex. VCH indicated that the total energy of MCC complex was less than that of LAC complex. This indicated that the drug was more soluble when combined with the microcrystalline complex. Molecular docking results also confirmed the dissolution result, in which the maximum drug release was seen with the MCC when compared with LAC. This was due to less H2 bonding present in the MCC in comparison to LAC.
Acknowledgments
The authors are thankful to the DST-FIST Laboratory of University Institute of Pharmaceutical Sciences, Panjab University, Chandigarh for providing instrumentation facilities.
Ethical approval
The Institutional Review Board approval is not required.
Declaration of patient consent
Patient’s consent not required as there are no patients in this study.
Conflicts of interest
There are no conflicts of interest.
Use of artificial intelligence (AI)-assisted technology for manuscript preparation
The authors confirm that there was no use of artificial intelligence (AI)-assisted technology for assisting in the writing or editing of the manuscript and no images were manipulated using AI.
Financial support and sponsorship
Nil.
References
- Drug-excipient compatibility studies: First step for dosage form development. Pharma Innov J. 2015;4:14-20.
- [Google Scholar]
- An overview of pharmaceutical excipients: Interactions and incompatibilities in dosage form development. Pharma Res J. 2022;1:65-72.
- [Google Scholar]
- A review on pharmaceutical preformulation studies in formulation and development of new drug molecules. Int J Pharm Sci Res. 2016;7:2313-20.
- [Google Scholar]
- Drug excipient compatibility studies in formulation development: Current trends and techniques. (AAPS) Formulation Design and Development (FDD) Section Newsletter 2015. p. :9-15.
- [Google Scholar]
- Preformulation studies: A versatile tool in formulation design. London, UK: IntechOpen; 2023.
- [CrossRef] [Google Scholar]
- Excipient applications in formulation design and drug delivery. In: Excipient applications in formulation design and drug delivery. Germany: Springer; 2015. p. :1-10.
- [CrossRef] [Google Scholar]
- ICH harmonized tripartite guideline impurities in new drug products Q3B(R2) 2006. Available from: https://www.ich.org/fileadmin/public_web_site/ICH_products/guidelines/quality/Q3B_R2/step4/Q3B_R2guideline.pdf [Last accessed on 2017 Sep 14]
- [Google Scholar]
- Interactions and incompatibilities of pharmaceutical excipients with active pharmaceutical ingredients: A comprehensive review. J Excip Food Chem. 2010;1:3-26.
- [CrossRef] [Google Scholar]
- Valacyclovir: A review of its antiviral activity, pharmacokinetic properties, and clinical efficacy. Antiviral Res. 1995;28:281-90.
- [CrossRef] [PubMed] [Google Scholar]
- Formulation and evaluation of valacyclovir hydrochloride effervescent floating tablets. Int J Adv Pharm Biotechnol. 2021;7:30-6.
- [CrossRef] [Google Scholar]
- Synthesis and characterization of valacyclovir HCl hybrid solid lipid nanoparticles by using natural oils. Recent Pat Drug Deliv Formul. 2019;13:46-61.
- [CrossRef] [PubMed] [Google Scholar]
- A study on the crystal transformation relationships of valacyclovir hydrochloride polymorphs: Sesquihydrate, form I, and form II. Cryst Res Technol. 2021;56:2100084.
- [CrossRef] [Google Scholar]
- A concise route to valacyclovir hydrochloride. Helv Chim Acta. 2011;94:592.
- [CrossRef] [Google Scholar]
- Comparative study of the selectivity power of colorimetric method over chromatographic methods for the analysis of valaciclovir hydrochloride. J AOAC Int. 2021;105:717-26.
- [CrossRef] [PubMed] [Google Scholar]
- Using autodock 4 and autodock vina with autodocktools: A tutorial USA: The Scripps Research Institute Molecular Graphics Laboratory; 2012. p. :92037.
- [Google Scholar]
- AutoDock Vina: Improving the speed and accuracy of docking with a new scoring function, efficient optimization, and multithreading. J Comput Chem. 2010;31:455-61.
- [CrossRef] [PubMed] [Google Scholar]
- PubChem substance and compound databases. Nucleic Acids Res. 2016;44:D1202-13.
- [CrossRef] [PubMed] [Google Scholar]
- GROMACS: Fast, flexible, and free. J Comput Chem. 2005;26:1701-18.
- [CrossRef] [PubMed] [Google Scholar]
- CHARMM36m: An improved force field for folded and intrinsically disordered proteins. Nat Methods. 2017;14:71-3.
- [CrossRef] [PubMed] [Google Scholar]
- The Lennard-Jones potential: when (not) to use it. Phys Chem Chem Phys. 2020;22:10624-33.
- [CrossRef] [PubMed] [Google Scholar]