Translate this page into:
Amplifying the human body’s innate “rapid response” systems to inflammation and oxidative stress
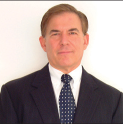
*Corresponding author: Mr. Bernie Landes, Nutritional Products Consulting Group LLC, Laguna Niguel, California. bernielandes@cox.net
-
Received: ,
Accepted: ,
How to cite this article: Landes B, Repine JE. Amplifying the human body’s innate “rapid response” systems to inflammation and oxidative stress. Am J Biopharm Pharm Sci 2022;2:8.
Inflammation is a key contributor to host defense against infection. A prime example is the rapid neutrophil response to bacterial infection during which neutrophils generate reactive oxygen species to kill ingested bacteria. While this powerful mechanism is vital to maintaining health, it can go awry and damage organs and tissues in the absence of infection. Ideally, the human body should have a remarkable ability to rapidly respond to and combat both inflammation and oxidative stress when it develops with or without the presence of infection. Inadvertent inflammation and oxidative stress may originate from a wide variety of metabolic, chemical, environmental, physical, disease, drug, and even emotional factors and at times when there is no infection. Unfortunately, the intrinsic ability of the body to rapidly respond to such challenges is limited in an increasingly significant number of individuals by certain genetic factors, metabolic dysfunction, dietary limitation, and lifestyle.
An important goal, therefore, is to restore optimal or near optimal functionality to critical mechanisms that counteract potentially detrimental inflammation and oxidative stress that occurs in the absence of infection. This concept forms an often overlooked foundational starting point as part of every rational program seeking to improve nutrition-based health and well-being.
Even in the most modern advanced countries, the potential to achieve optimal health by improving the responses to inflammation and oxidative stress is either limited or virtually absent. Moreover, regrettably, nutritional programs are based more on “the next big thing” rather than establishing a strong foundation based on organically or biodynamically grown and minimally processed nutrient-dense whole foods coupled with targeted supplementation. This type of supplementation is likely needed in certain individuals. Appropriately and rationally based supplementation that focuses on three limited or missing elements that when provided in unison and on a consistent basis could support and amplify the body’s rapid response to inflammation and oxidative stress deserves consideration.
The critical importance of the multiple body “systems” that must function both as separate systems and in harmony with all the other body systems to maintain a fully functional organism is well recognized. The entire human biological system includes cardiovascular, respiratory, endocrine, reproductive, digestive, immune, and nervous systems. No system is one dimensional and no system functions independently. Absent the coordination of these systems, we cease to be able to continue living. Moreover, of course, there is no such thing as the “next big system.” That is why recognition, restoration, and maintenance of the “rapid response system” that provides the ability to deal effectively with both inflammation and oxidative stress caused by a myriad of environmental, physiological, pathological, and emotional challenges emerges as a vitally important consideration. Further, it is also why approaches relying on a dietary supplement program that relies on single compounds in a never-ending variety are essentially limited at best as the needed way to provide sufficient protection against excessive inflammation and oxidative stress [Figure 1].

- Amplifying the human body’s innate “rapid response” systems to inflammation and oxidative stress.
The purpose of this brief opinion is to identify critical elements that can respond rapidly to an acute inflammatory and oxidative stress response and to suggest how these key elements can be supplemented in unison to improve the efficacy of any one pathway individually. This review will focus on three nutritional elements – ascorbate, L-ergothioneine, and glutathione.
First, the ability to produce ascorbate (Vitamin C) [Figure 2] in the human liver in response to oxidative stress and inflammation is absent in humans due to a genetic anomaly that makes l-gulonolactone oxidase unavailable to transform glucose in the liver. This enzyme deficiency is a suspiciously problematic genetic characteristic of humans since this enzyme deficiency is common only in a very small number of animals (monkeys, apes, fruit bats, guinea pigs, and a few varieties of birds). At present, approaches to optimize the availability of ascorbate in humans, including the recommended level of daily intake set forth by the U.S. Government, focus only on providing sufficient Vitamin C, with an added safety factor, to protect against the development of scurvy. Approaches that profess to provide benefit from higher levels of ingested ascorbic acid are questioned with respect to safety and efficacy for the claimed benefits. This impression is based in part on the intermittent requirement for ascorbate by the body and in part because the acid form of ascorbate must be neutralized by a variety of compounds, including minerals, before efficient use by the body.[1-23]

- Chemical structure of Ascorbic Acid.
Next, the human body, by design, has a unique gene (SLC22A4) that is specifically responsible for the utilization of a unique sulfur compound called L-ergothioneine [Figure 3]. This gene codes for an ergothioneine transporter, OCTN1, which is produced in response to an increase in oxidative stress and inflammation that exceeds the body’s ability to respond with available antioxidant resources, including vitamins and plant-derived polyphenols. Ergothioneine is a unique molecule, first discovered in 1909, that is produced in the soil by microorganisms, including fungi and bacteria, as part of nature’s natural recycling of deteriorated plant and animal matter. Neither plants nor animals can produce it. Ergothioneine can only be passed on by way of what is produced by these microorganisms and taken up by plants that pass it on directly to animals or humans. Accordingly, plants then pass it on to animals and, in turn, then to humans. As a result, and because of the nature of the typical American diet, the amount of ergothioneine consumed is highly variable and either extremely limited or absent altogether. Ergothioneine has some unique characteristics. For example, it acts as an independent antioxidant, but may also amplify and extend the activity of other antioxidants, including Vitamin C and Vitamin E. The half-life of ergothioneine is 30 days, compared to only minutes or seconds of other antioxidants. Notably, ergothioneine has been suggested to be an essential vitamin by leading scientific authorities, including Dr. Bruce Ames and Dr. Solomon Snyder. Ergothioneine is concentrated in humans in the brain, eyes, kidneys, skin, and the reproductive organs – ostensibly sites that are particularly prone to damage from inflammation and oxidative stress. Further, the relatively little amount of ergothioneine that is present in younger individuals diminishes with age, a phenomenon that has prompted some experts in the scientific community to suggest a role for ergothioneine in the aging process.[24-30]

- Chemical structure of L-Ergothioneine.
Finally, glutathione is known as the body’s “master antioxidant” and a key component of the glutathione redox cycle. Like ergothioneine, glutathione is a sulfur-containing molecule present in every cell of the body. Human cells are designed to maintain glutathione homeostasis as the third part of the body’s rapid response to oxidative stress. Glutathione is produced by a series of enzymatic actions that initially link the amino acids cysteine and glutamate and then ultimately to the amino acid glycine. This process requires not only adequate levels of the three amino acids but also a fully functioning suite of enzymes at every stage of the process. Most importantly, glutathione in its finished form cannot be taken up by the cells of the body due to an unfavorable concentration gradient. Rather, cells rely on a constant internal supply of the immediate precursor, gamma-glutamylcysteine (GGC) [Figure 4]. GGC is then linked to glycine in a continuously operating system designed to maintain glutathione homeostasis in response to oxidative stress and inflammation. Unfortunately, for a variety of reasons, including most notably aging, inadequate levels of glutathione develop, most likely because of deficiencies in the required enzymes and dietary limitations. Consequently, the homeostatic glutathione maintenance system may not be fully functional, leaving the body at elevated risk of a variety of health challenges directly related to unresolved oxidative stress and inflammation. GGC is present in a small number of foods, such as undenatured (heat processed) whey, but is impossible to obtain from the diet in a clinically useful amount. In addition, consuming pre-formed glutathione is an inefficient way to raise cellular levels of glutathione since published clinical studies demonstrate that it may take weeks or months to impact cellular levels when elevated levels are most needed acutely.[31-43]
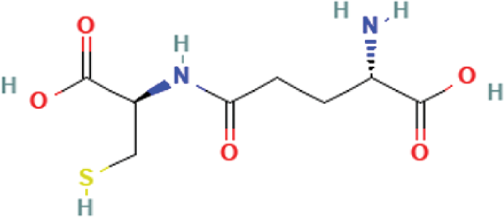
- Chemical structure of Gamma-glutamylcysteine.
While each of these compounds is individually available and known to have an established safety profile, administration of any one of these, and even more so, taking them in unison in a timely fashion is rarely, if ever, incorporated into a clinical regimen or daily supplement regimen. Significantly, the combination is never taken together during an unwarranted inflammation or oxidative stress exacerbation. Perhaps, this concept should be considered and tested using this approach in conjunction with measuring blood biomarkers that reflect individual and pathway proteins that correspond to inflammation and oxidative stress.
Taken together, this combination of dietary supplements could represent the first opportunity for humans to potentially and simultaneously activate three critical rapid response capabilities in unison that combat inflammation and oxidative stress. Moreover, because of the detrimental effects of inflammation and oxidative stress and reductions in these and other anti-inflammatory and antioxidant protective mechanisms during aging, supplementing one’s diet on a daily basis with all three of these agents as part of a health maintenance regimen might be warranted and well advised. A potential concern is whether amplifying this rapid response system in this way could limit host defense mechanisms that are needed intermittently during a response to infection. However, that potential concern should be evaluated as part of a continuing research focus on addressing the negative and potentially catastrophic effects of out of control inflammation and oxidative stress when it is not needed for host defense.
References
- Effect of Vitamin C infusion on organ failure and biomarkers of inflammation and vascular injury in patients with sepsis and severe acute respiratory failure: The CITRISALI randomized clinical trial. JAMA. 2019;322:1261-70.
- [CrossRef] [PubMed] [Google Scholar]
- Vitamin C and immune cell function in inflammation and cancer. Biochem Soc Trans. 2018;46:1147-59.
- [CrossRef] [PubMed] [Google Scholar]
- Vitamin C: Should we supplement? Curr Opin Crit Care. 2018;24:248-55.
- [CrossRef] [PubMed] [Google Scholar]
- The long history of Vitamin C: From prevention of the common cold to potential aid in the treatment of COVID-19. Front Immunol. 2020;11:574029.
- [CrossRef] [PubMed] [Google Scholar]
- Vitamin C alleviates hyperuricemia nephropathy by reducing inflammation and fibrosis. J Food Sci. 2021;86:3265-76.
- [CrossRef] [PubMed] [Google Scholar]
- Feasibility of Vitamin C in the treatment of post viral fatigue with focus on long COVID, based on a systematic review of IV Vitamin C on fatigue. Nutrients. 2021;13:1154.
- [CrossRef] [PubMed] [Google Scholar]
- Effects of Vitamin C on oxidative stress, inflammation, muscle soreness, and strength following acute exercise: Meta-analyses of randomized clinical trials. Eur J Nutr. 2020;59:2827-39.
- [CrossRef] [PubMed] [Google Scholar]
- Vitamin C: The next step in sepsis management? J Crit Care. 2018;43:230-4.
- [CrossRef] [PubMed] [Google Scholar]
- Obesity, cardiovascular disease, and role of Vitamin C on inflammation: A review of facts and underlying mechanisms. Inflammopharmacology. 2017;25:313-28.
- [CrossRef] [PubMed] [Google Scholar]
- Vitamin C: A review on its role in the management of metabolic syndrome. Int J Med Sci. 2020;17:1625-38.
- [CrossRef] [PubMed] [Google Scholar]
- Inflammation in the vascular bed: Importance of Vitamin C. Pharmacol Ther. 2008;119:96-103.
- [CrossRef] [PubMed] [Google Scholar]
- Preventive and therapeutic potential of Vitamin C in mental disorders. Curr Med Sci. 2018;38:1-10.
- [CrossRef] [PubMed] [Google Scholar]
- Effects of Vitamin C on health: A review of evidence. Front Biosci (Landmark Ed). 2013;18:1017-29.
- [CrossRef] [PubMed] [Google Scholar]
- Effects of citrus fruit juices and their bioactive components on inflammation and immunity: A narrative review. Front Immunol. 2021;12:712608.
- [CrossRef] [PubMed] [Google Scholar]
- Prooxidative inhibition against NF-κB-mediated inflammation by pharmacological vitamin C. Free Radic Biol Med. 2022;180:85-94.
- [CrossRef] [PubMed] [Google Scholar]
- Associations of Vitamin C status, fruit and vegetable intakes, and markers of inflammation and hemostasis. Am J Clin Nutr. 2006;83:567-74.
- [CrossRef] [PubMed] [Google Scholar]
- Effect of Vitamin C on endothelial function in health and disease: A systematic review and meta-analysis of randomised controlled trials. Atherosclerosis. 2014;235:9-20.
- [CrossRef] [PubMed] [Google Scholar]
- Hypovitaminosis C and Vitamin C deficiency in critically ill patients despite recommended enteral and parenteral intakes. Crit Care. 2017;21:300.
- [CrossRef] [PubMed] [Google Scholar]
- Effect of Vitamin C supplementation on lipid peroxidation, muscle damage and inflammation after 30-min exercise at 75% VO2max. J Sports Med Phys Fitness. 2008;48:217-24.
- [Google Scholar]
- Serum Vitamin C concentration is low in peripheral arterial disease and is associated with inflammation and severity of atherosclerosis. Circulation. 2001;103:1863-8.
- [CrossRef] [PubMed] [Google Scholar]
- Diet as a hot topic in psychiatry: A population-scale study of nutritional intake and inflammatory potential in severe mental illness. World Psychiatry. 2018;17:365-7.
- [CrossRef] [PubMed] [Google Scholar]
- Ergothioneine-a diet-derived antioxidant with therapeutic potential. FEBS Lett. 2018;592:3357-66.
- [CrossRef] [PubMed] [Google Scholar]
- Ergothioneine, recent developments. Redox Biol. 2021;42:101868.
- [CrossRef] [PubMed] [Google Scholar]
- The biology of ergothioneine, an antioxidant nutraceutical. Nutr Res Rev. 2020;33:190-217.
- [CrossRef] [PubMed] [Google Scholar]
- The unusual amino acid L-ergothioneine is a physiologic cytoprotectant. Cell Death Differ. 2010;17:1134-40.
- [CrossRef] [PubMed] [Google Scholar]
- L-ergothioneine modulates oxidative damage in the kidney and liver of rats in vivo: Studies upon the profile of polyunsaturated fatty acids. Clin Nutr. 2004;23:183-93.
- [CrossRef] [Google Scholar]
- Free radicals, oxidative stress, and antioxidants in human health and disease. J Am Oil Chem Soc. 1998;75:199-212.
- [CrossRef] [PubMed] [Google Scholar]
- γ-Glutamylcysteine detoxifies reactive oxygen species by acting as glutathione peroxidase-1 cofactor. Nat Commun. 2012;3:718.
- [CrossRef] [PubMed] [Google Scholar]
- γ-Glutamylcysteine alleviates ischemic stroke-induced neuronal apoptosis by inhibiting ROS-mediated endoplasmic reticulum stress. Oxid Med Cell Longev. 2021;2021:2961079.
- [CrossRef] [PubMed] [Google Scholar]
- γ-glutamylcysteine exhibits anti-inflammatory effects by increasing cellular glutathione level. Redox Biol. 2019;20:157-66.
- [CrossRef] [PubMed] [Google Scholar]
- Supplementation with γ-glutamylcysteine (γ-GC) lessens oxidative stress, brain inflammation and amyloid pathology and improves spatial memory in a murine model of AD. Neurochem Int. 2021;144:104931.
- [CrossRef] [PubMed] [Google Scholar]
- Glutathione and γ-glutamylcysteine in the antioxidant and survival functions of mitochondria. Biochem Soc Trans. 2013;41:106-10.
- [CrossRef] [PubMed] [Google Scholar]
- Mitochondrial glutathione and oxidative stress: Implications for pulmonary oxygen toxicity in premature infants. Mol Genet Metab. 2000;71:352-8.
- [CrossRef] [PubMed] [Google Scholar]
- γ-Glutamylcysteine synthetase (γ-GCS) as a target for overcoming chemo-and radio-resistance of human hepatocellular carcinoma cells. Life Sci. 2018;198:25-31.
- [CrossRef] [PubMed] [Google Scholar]
- Gamma-glutamylcysteine protects ergothioneine-deficient Mycobacterium tuberculosis mutants against oxidative and nitrosative stress. Biochem Biophys Res Commun. 2018;495:174-8.
- [CrossRef] [PubMed] [Google Scholar]
- Glutamate cysteine ligase and the age-related decline in cellular glutathione: The therapeutic potential of γ-glutamylcysteine. Arch Biochem Biophys. 2016;593:12-23.
- [CrossRef] [PubMed] [Google Scholar]
- Protective effect of γ-glutamylcysteine against UVB radiation in NIH-3T3 cells. Photodermatol Photoimmunol Photomed 2022 Epub ahead of print 10.1111/phpp.12782
- [CrossRef] [PubMed] [Google Scholar]
- γ-Glutamylcysteine inhibits oxidative stress in human endothelial cells. Life Sci. 2012;90:116-21.
- [CrossRef] [PubMed] [Google Scholar]
- Oral administration of γ-glutamylcysteine increases intracellular glutathione levels above homeostasis in a randomized human trial pilot study. Redox Biol. 2017;11:631-6.
- [CrossRef] [PubMed] [Google Scholar]
- Dietary γ-glutamylcysteine: Its impact on glutathione status and potential health outcomes. J Diet Suppl. 2022;19:259-70.
- [CrossRef] [PubMed] [Google Scholar]