Translate this page into:
Alpha-tocopherol and quercetin modulate primary hemodynamic parameters, oxidative stress indices, and biomarkers of cardio-renal functioning in ethanol-exposed rats
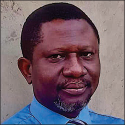
*Corresponding author: Oluwaseun Esan, Department of Veterinary Medicine, University of Ibadan, Ibadan, Oyo, Nigeria. esan.seun2014@gmail.com
-
Received: ,
Accepted: ,
How to cite this article: Ajibade TO, Oyetunji GB, Esan O, Adetona M, Oyagbemi A, Omobowale T, et al. Alpha-tocopherol and quercetin modulate primary hemodynamic parameters, oxidative stress indices, and biomarkers of cardio-renal functioning in ethanol-exposed rats. Am J Biopharm Pharm Sci. 2024;4:6. doi: 10.25259/AJBPS_6_2024
Abstract
Objectives:
The objective of this study was the assessment of the ameliorative roles of alpha-tocopherol and quercetin on the toxic mechanisms associated with ethanol (EtoH) exposure in the cardiac and renal systems of rats.
Material and Methods:
Forty male rats were randomly selected and divided into five groups as follows: 0.2 mL distilled water; EtoH 40% v/v; EtoH + 2.5 mg/kg alpha-tocopherol; EtoH + 50 mg/kg quercetin; and EtoH + a cocktail of alpha-tocopherol and quercetin.
Results:
Treatment with alpha-tocopherol and quercetin significantly (P < 0.05) ameliorated EtoH-induced alterations in hemodynamic and electrocardiographic parameters, kidney function markers, and antioxidant defense status of rats with significantly elevated levels of glutathione, glutathione peroxidase, superoxide dismutase, and glutathione-S-transferase observed in antioxidant-treated rats. Histopathologic lesions induced by EtoH including focal loss of myofiber striation, degeneration, and infiltration of inflammatory cells in the cardiac tissues, as well as patchy tubular necrosis, congestion, and ectasia in renal tissues were absent in the antioxidant treated rats. Heightened immunohistochemical expressions of cardiac-specific troponin and angiotensin converting enzymes induced by EtoH were abated by alpha-tocopherol and quercetin treatment.
Conclusion:
Alpha-tocopherol and quercetin mitigated oxidative stress-mediated ethanol-induced derangements of the cardiovascular and renal systems in rats.
Keywords
Alpha-tocopherol
Quercetin
Ethanol
Necrosis
Troponin
INTRODUCTION
The exposure to ethanol (EtoH) has continued to pose significant global public health concerns, with excessive EtoH intake unequivocally associated with an increased risk of cardiovascular disease.[1,2] Overwhelming increases have been recorded in the rates of alcohol consumption, with skyrocketing implications on health and general well-being, and positive correlation reported between increased alcohol consumption, all-cause mortality, and cancers.[3,4] Unfortunately, moderate to high exposure induces complex changes in blood biochemistry and alterations in many biomarkers for cardiometabolic risk.[5,6] Furthermore, alcohol exposure has been positively correlated with incidences of cardiomyopathy, high blood pressure, increased risk of myocardial infarction, arrhythmias, fatal cardiac arrest, and stroke.[7]
Epidemiologic, clinical, and experimental reports abound in literature to underline the compelling evidence for alcohol-induced hypertension.[8] Moreover, alcohol-induced heightened blood pressure parameters have been linked with the potentiation of the renin-angiotensin aldosterone system with consequent increases in peripheral resistance, expansion of blood volume, and induction of oxidative stress in vascular endothelium.[9,10] In the same vein, irreversible toxicological changes and loss of renal tubular functioning have been associated with excessive EtoH exposure,[11] through the potentiation of oxidative processes on proteins and deoxyribonucleic acid in the kidneys.[12,13]
Antioxidants such as chrysin, phenolic acids, and flavonoids, as well as antioxidant-rich medicinal plants such as Panax ginseng and Silybum maximum have been reported to significantly mitigate oxidative-stress-mediated tissue damage associated with alcohol exposure.[13,14] Alpha-tocopherol and quercetin are potent dietary antioxidants.[14,15] Alpha-tocopherol, a fat-soluble non-enzymatic antioxidant, inhibits lipid peroxidation reaction and prevents oxidative damage in cell membranes of various mammalian tissues.[16-21] Quercetin occurs naturally in many fruits and vegetables and has been reported to scavenge free radicals and mitigate pathogenic oxidative mechanisms in vivo.[22] The objective of this study was the assessment of the ameliorative roles of alpha-tocopherol and quercetin on the toxic mechanisms associated with EtoH exposure in the cardiac and renal systems of rats.
MATERIAL AND METHODS
Experimental animals
Following an acclimatization period of 2 weeks, Groups 1–5 of eight animals each were assigned as follows: Control; EtoH 40% v/v, 5 g/kg;[23] EtoH and alpha-tocopherol 2.5 mg/kg;[24] EtoH and quercetin 50 mg/kg;[25] and EtoH and a cocktail of alpha-tocopherol/quercetin. Alcohol and quercetin were dissolved in distilled water, whereas alpha-tocopherol was dissolved in corn oil. The administration period lasted for 40 consecutive days.
Ethical approval
The experiment was carried out in accordance with ethical standards laid down by the University of Ibadan Animal Care Use and Research Ethics Committee with the approval number UI-ACUREC/TOA/20/0036.
Primary hemodynamic parameters
The primary hemodynamic parameters are as follows: Mean, diastolic and systolic blood pressures, and heart rate were monitored in non-anesthetized rats with a plethysmograph (CODA, Kent scientific), whereas indices of cardiac performance including the P-wave and QRS duration, QT interval, and R wave amplitude were obtained with an electrocardiograph.[26]
Preparation of serum and tissues for biochemical assays
The heart and kidney samples were harvested and kept at −4°C immediately till the time of analysis. The serum was also collected after the blood samples were clot and stored immediately at −4°C.
Homogenization
Kidney and heart tissues, from freshly sacrificed rats were homogenized, in 0.1 M phosphate buffer, with a Teflon homogenizer, and the resulting homogenate was centrifuged in a cold centrifuge at 10,000 rpm for 10 min to obtain post-mitochondrial fraction used for analysis of the markers of oxidative stress and systemic antioxidants.
Analysis oxidative stress markers and systemic antioxidants
The assay for oxidative damage in cardiac and renal tissues was determined as previously described.[27] The thiol contents and reduced glutathione (GSH) level were assayed as previously described,[28] whereas hydrogen peroxide generation and superoxide dismutase (SOD) level was measured as described by Wolff.[29] and Misra and Fridovich,[30] respectively. Glutathione S-transferase (GST), glutathione peroxidase (GPx), nitric oxide (NO), and myeloperoxidase (MPO) were assayed in accordance with the methods Habig et al.,[31] Beutler et al.,[32] Miranda et al.,[33] and Xia and Zweierm,[34] respectively. The blood urea nitrogen (BUN) and creatinine were determined using standard methods as highlighted in the manufacturer’s instructions on their respective kits (Randox Ltd, UK).
Preparation of tissues for histopathological and immunohistochemical evaluation
Small pieces of kidney and heart were fixed in 10% formalin, embedded in paraffin wax, and sections of 5–6 mm in thickness were made and, thereafter, stained with hematoxylin and eosin for histopathological examination according to the methods as previously described.[35] The tissues were subsequently used to evaluate the immunohistochemical expressions of cardiac specific troponin I (cTn I) in renal tissues and angiotensin-converting enzyme (ACE) in renal tissues of rats.
Statistical analysis
The data obtained from the experimental animals were analyzed using descriptive statistics. Analysis of variance with Dunnett’s post-test was used to analyze statistical significance difference in the groups. A statistical package (Prism 8.0) was used to analyze the data.
RESULTS
The result of the effects of the administered antioxidants (alpha-tocopherol and quercetin) on alcohol-induced alterations of arterial pressures and electrocardiographic changes is presented in Tables 1 and 2, respectively. Alcohol altered the hemodynamic parameters, that is, the arterial pressures and heart rate, in comparison with control, whereas alpha-tocopherol and quercetin significantly ameliorated the alcohol-induced alterations, with near-normal values recorded with the coadministration of alpha-tocopherol and quercetin. Furthermore, alcohol induced a significant decrease in PR and QT intervals, and the duration of QRS complex relative to alpha-tocopherol and quercetin treated rats.
Parameter | Control | EtoH | EtoH+α+T | EtoH+Q | EtoH+α+T+Q |
---|---|---|---|---|---|
Systolic BP | 121.5±3.2 | 186.6±1.3a | 141.6±2.1b | 149.6±4.8b | 115.5±3.4c |
Diastolic BP | 93.5±2.6 | 148.0±5.6a | 95.6±2.7b | 104.8±5.9b | 81.18±1.7c |
Mean BP | 104.0±2.36 | 160.8±3.8a | 110.8±1.7b | 118.2±5.3b | 92.4±2.0c |
Heart rate | 352.5±10.5 | 375.2±11.9a | 392.8±10.7b | 364.3±9.1b | 381.8±12.2c |
Blood pressure (BP) measured in millimeter of mercury (mmHg), Heart rate measured in beats per minute. EtoH=Ethanol (40%); α-T=alpha-tocopherol (2.5 mg/kg); Q=quercetin (50 mg/kg). asignificant (p<0.05) difference compared with control, bsignificant (p<0.05) difference compared with ethanol (EtOH), csignificant (p<0.05) difference compared with EtoH+Q.
Parameter | Control | EtoH | EtoH+α+T | EtoH+Q | EtoH+α+T+Q |
---|---|---|---|---|---|
P wave (ms) | 20.1±0.3 | 21.3±1.4 | 20.4±1.2 | 21.2±0.7 | 19.8±1.2 |
PR interval (ms) | 33.4±1.2 | 29.8±1.2a | 31.4±1.2 | 30.4±1.2 | 32.8±1.6 |
QT interval (ms) | 96.0±6.1 | 62.4±3.2a | 80.5±7.2b | 76.4±3.8b | 92.1±8.3b |
QRS duration (ms) | 18.2±1.3 | 12.7±4.6a | 14.3±2.1b | 15.3±1.8 | 17.5±2.1b |
QTC (ms) | 121.6±3.4 | 138.4±6.2a | 92.1±3.7 | 111.4±2.5 | 108.4±2.9 |
EtoH=Ethanol (40%); α-T=alpha-tocopherol (2.5 mg/kg); Q=quercetin (50 mg/kg). P wave = duration of atrial depolarization; PR interval = duration of atrial depolarization and delay of impulse conduction in the atrioventricular node; QT interval = duration of ventricular depolarization and repolarization; QRS duration = total time for the depolarization of all parts of the ventricle; QTC = corrected QT interval. asignificant (p<0.05) difference compared with control. bsignificant (p<0.05) difference compared with ethanol (EtOH).
The result of the effects of the administered antioxidants (alpha-tocopherol and quercetin) on alcohol-induced alterations of markers of oxidative stress in cardiac and renal tissues of rats is presented in Figures 1-4. Alcohol induced a significant elevation of hydrogen peroxide, malondialdehyde (MDA), and sulfhydryl thiol content, but the opposite effect was seen with the analyzed systemic antioxidants reduced GSH, GPx, SOD, and GST [Figures 5-8]. However, alpha-tocopherol and quercetin significantly ameliorated these effects, with near to normal levels of oxidative stress markers recorded in rats administered both alpha-tocopherol and quercetin. In addition, the levels of serum MPO, urea, and creatinine were significantly increased by antioxidant treatment [Figures 9-11].
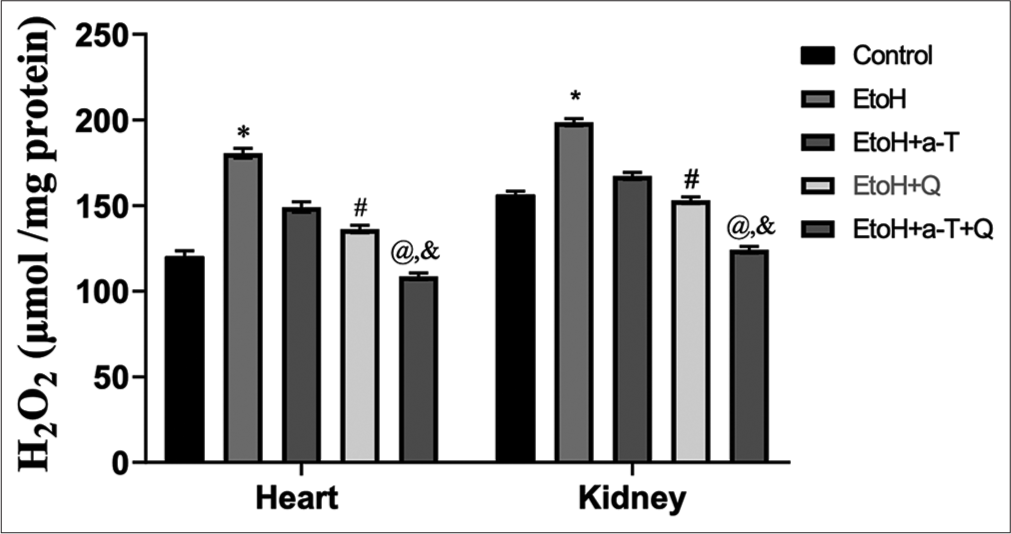
- Hydrogen peroxide level in cardiac and renal tissues. Superscript (*) indicates significant (P < 0.05) increase compared with control; # indicates significant (P < 0.05) decrease compared with ethanol (EtoH); @ indicates significant (P < 0.05) decrease compared with EtoH+α-T; ‘&’ indicates significant (P < 0.05) decrease compared with EtoH+Q.
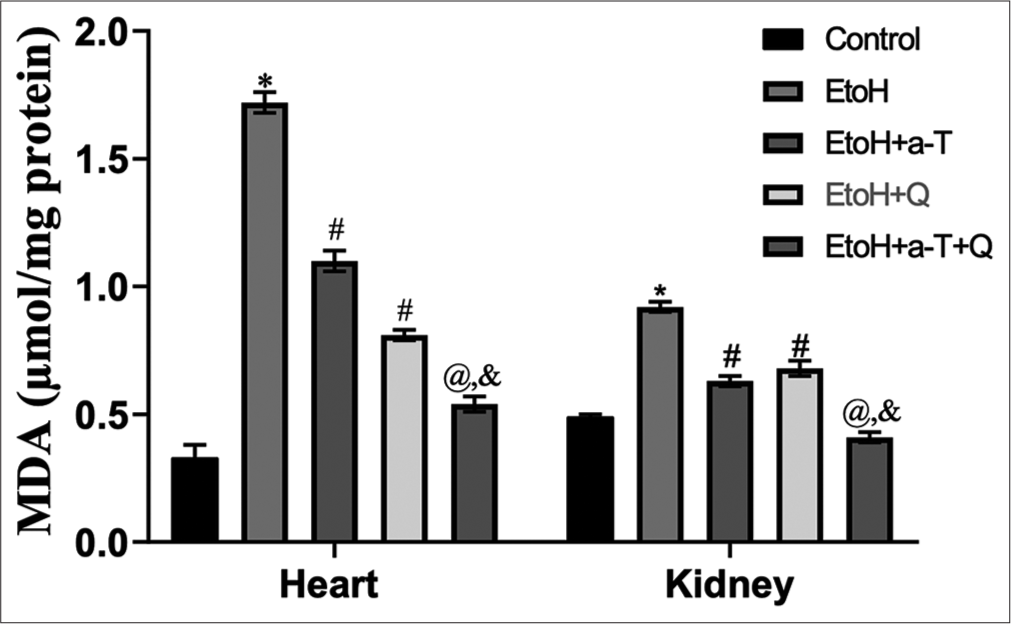
- Malondialdehyde level in cardiac and renal tissues. Superscript (*) indicates significant (P < 0.05) increase compared with control; # indicates significant (P < 0.05) decrease compared with ethanol (EtoH); @ indicates significant (P < 0.05) decrease compared with EtoH + α-T; ‘&’ indicates significant (P < 0.05) decrease compared with EtoH+Q. MDA: malondialdehyde.
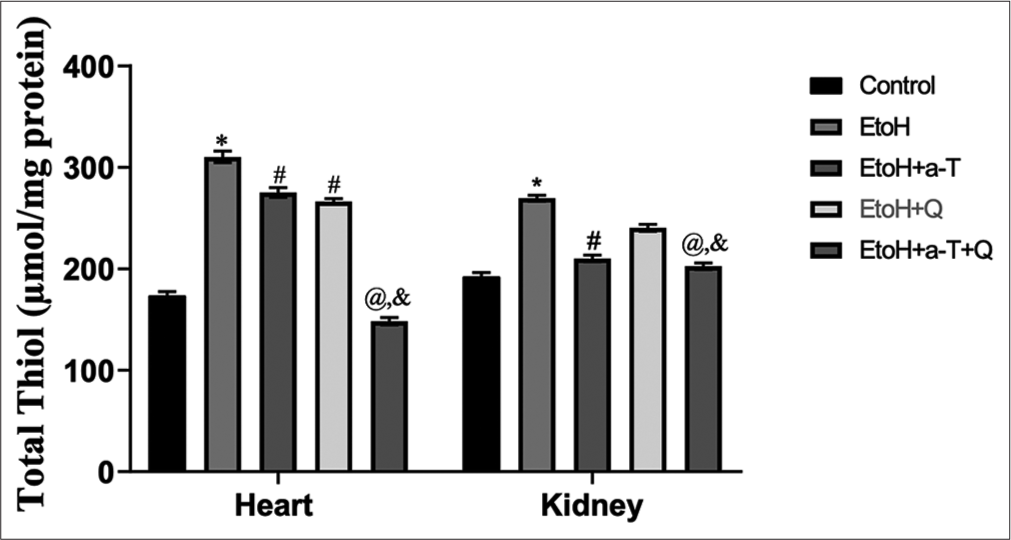
- Total Tthiol level in cardiac and renal tissues. Superscript (*) indicates significant (P < 0.05) increase compared with control; # indicates significant (P < 0.05) decrease compared with ethanol (EtoH); @ indicates significant (P < 0.05) decrease compared with EtoH+α-T; ‘&’ indicates significant (P < 0.05) decrease compared with EtoH+Q.
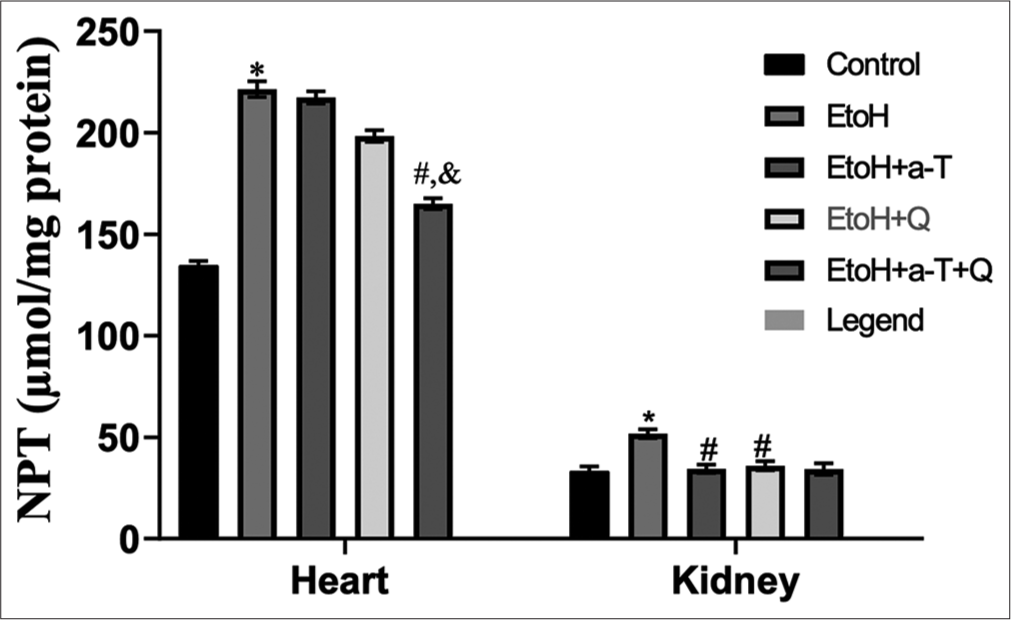
- Non-protein thiol (NPT) in cardiac and renal tissues. Superscript (*) indicates significant (P < 0.05) increase compared with control; # indicates significant (P < 0.05) decrease compared with ethanol (EtoH); @ indicates significant (P < 0.05) decrease compared with EtOH+α-T; ‘&’ indicates significant (P < 0.05) decrease compared with EtoH+Q.
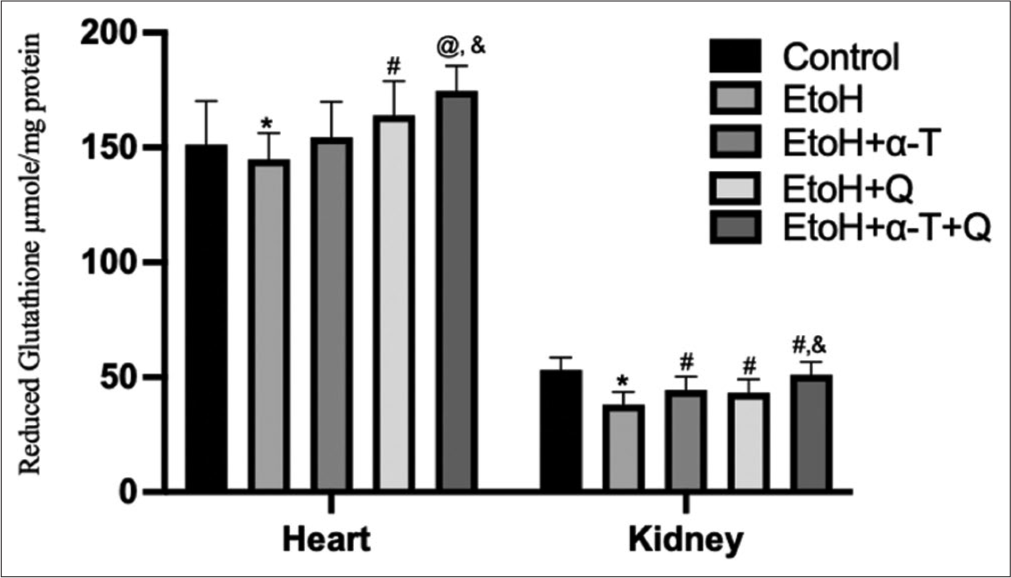
- Reduced glutathione level in cardiac and renal tissues of rats. Superscript “*” indicates significant (P < 0.05) decrease compared with control; Superscript “#” indicates significant (P < 0.05) increase compared with ethanol (EtOH); Superscript “@” indicates significant (P < 0.01) increase compared with EtOH Superscript “&” indicates significant (P < 0.05) increase compared with EtoH+Q.
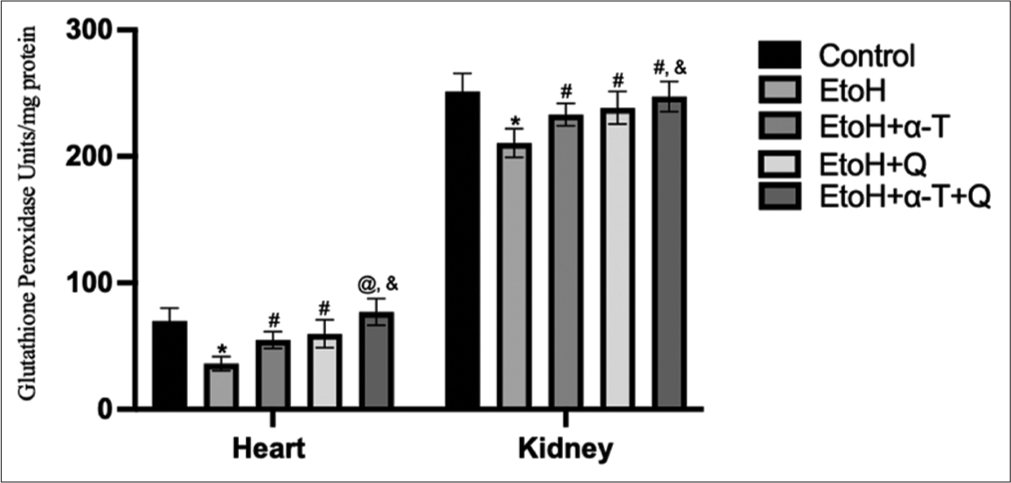
- Glutathione peroxidase activity in cardiac and renal tissues of rats. Superscript “*” indicates significant (P < 0.01) decrease compared with control; Superscript “#” indicates significant (P < 0.05) increase compared with ethanol (EtOH); Superscript “@” indicates significant (P < 0.01) increase compared with EtOH Superscript “&” indicates significant (P < 0.05) increase compared with EtoH+Q.
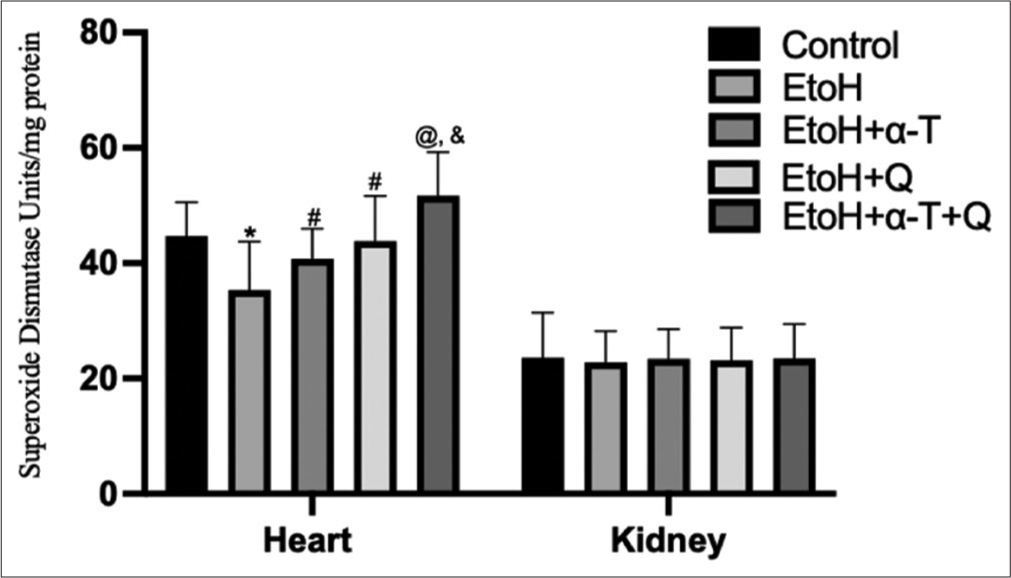
- Superoxide dismutase activity in cardiac and renal tissues of rats. Superscript “*” indicates significant (P < 0.01) decrease compared with control; Superscript “#” indicates significant (P < 0.05) increase compared with ethanol (EtOH); Superscript “@” indicates significant (P < 0.01) increase compared with EtOH; Superscript “&” indicates significant (P < 0.05) increase compared with EtoH+Q.
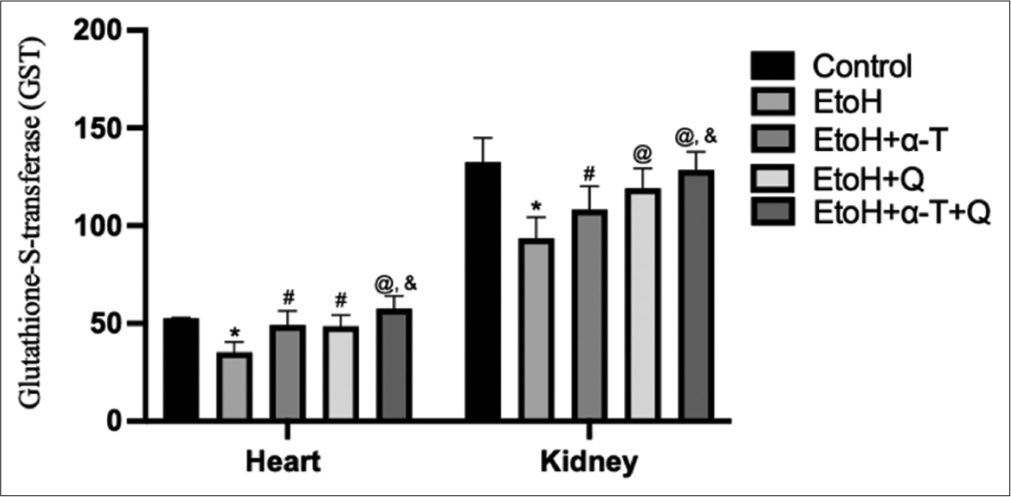
- Glutathione (GSH)-S-transferase (mmole l-chloro-2, 4 dinitrobenzene-GSH complex formed/min/mg protein) activity in cardiac and renal tissues of rats. Superscript “*” indicates significant (P < 0.01) decrease compared with control; Superscript “#” indicates significant (P < 0.05) increase compared with ethanol (EtoH); Superscript “@” indicates significant (P < 0.01) increase compared with EtOH; Superscript “&” indicates significant (P < 0.05) increase compared with EtoH+Q.
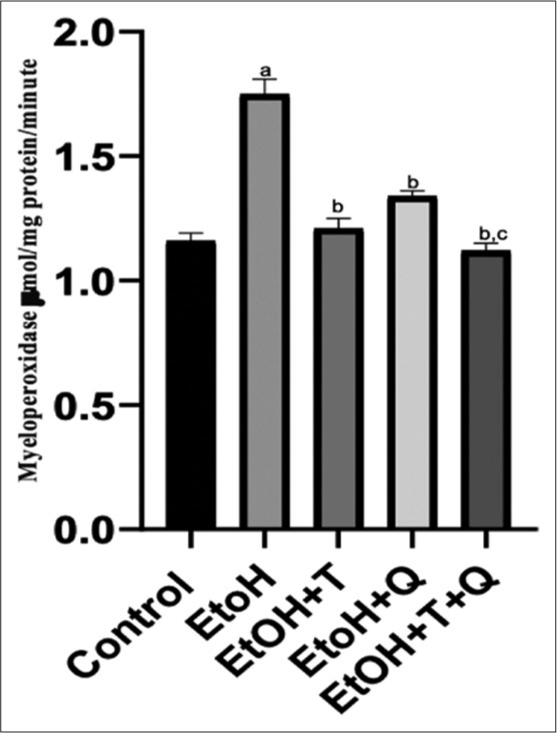
- Alcohol-induced alteration of serum myeloperoxide and effect of antioxidants. Superscript a indicates significant (P < 0.05) increase compared with control; Superscript b indicates significant (P < 0.05) decrease compared with ethanol (EtoH); Superscript c; indicates significant decrease compared with EtOH+Q.
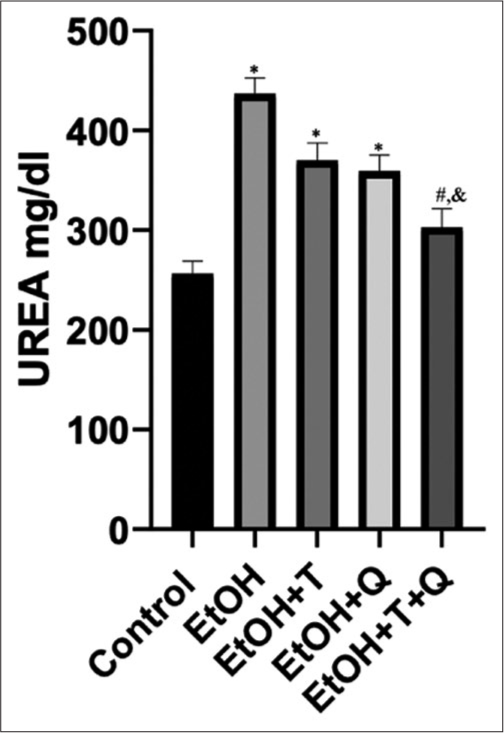
- Alcohol-induced changes in urea concentration and effects of antioxidants. Superscript (*) indicates significant increase compared with control; Superscript (#) indicates significant decrease compared with ethanol (EtoH); Superscript (&) indicates significant decrease compared with EtoH+Q.
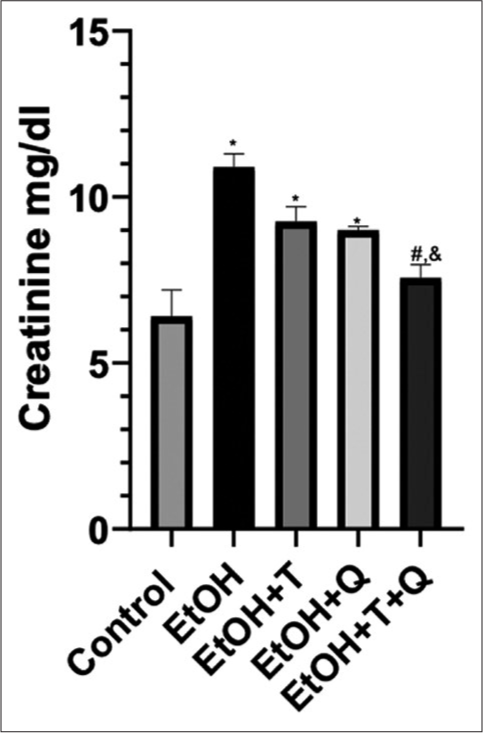
- Alcohol-induced changes in creatinine concentration and effects of antioxidants. Superscript (*) indicates significant increase compared with control; Superscript (#) indicates significant decrease compared with ethanol (EtoH); Superscript (&) indicates significant decrease compared with EtoH+Q.
Histopathologic lesions induced by EtoH include focal loss of myofiber striation, degeneration, and infiltration of inflammatory cells in the cardiac tissues, as well as patchy tubular necrosis, congestion, and ectasia in renal tissues. These were absent in the antioxidant treated rats [Figures 12 and 13]. Heightened immunohistochemical expressions of cTn [Figure 14] and ACEs [Figure 15] induced by EtoH were abated by alpha-tocopherol and quercetin treatment.
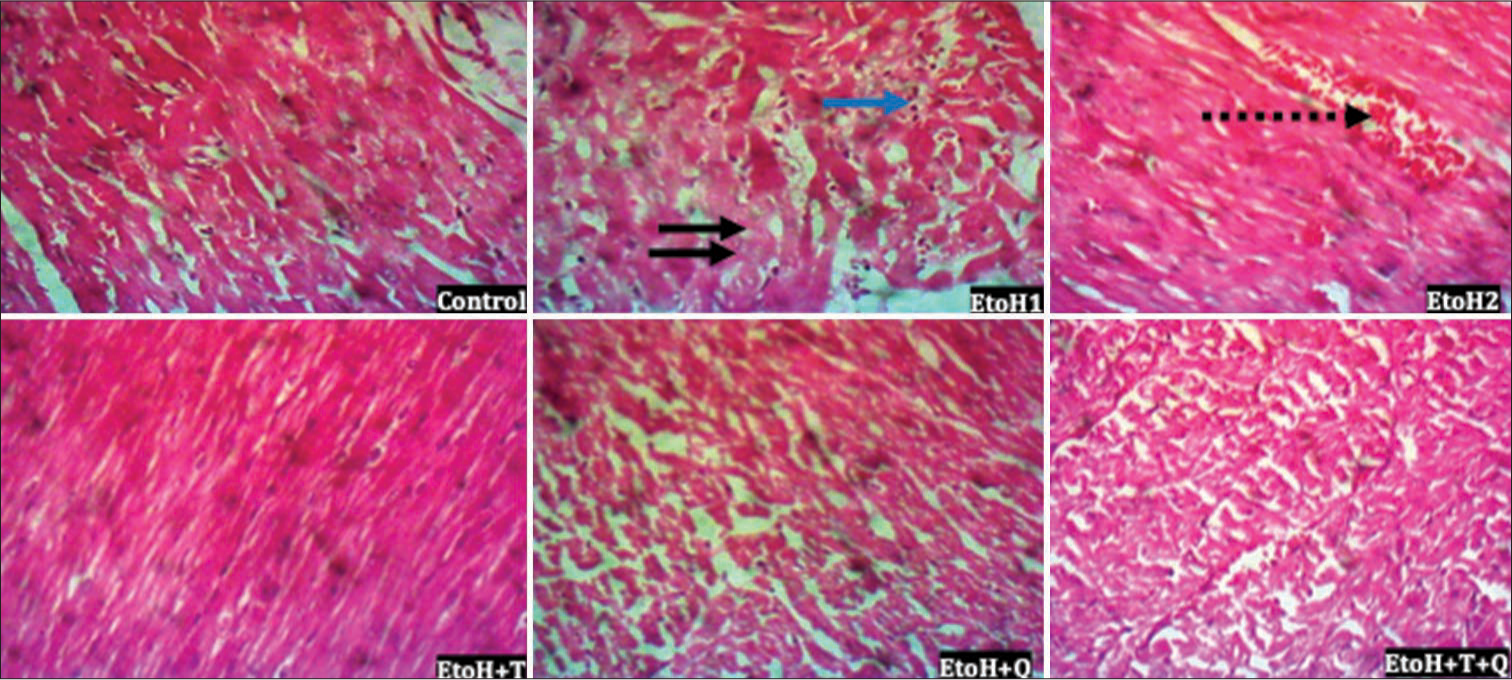
- Photomicrograph of cardiac tissue showing focal loss of myofiber striation, degeneration (black arrows), a few infiltrate of inflammatory cells (blue arrow) and congestion (broken arrow); hematoxylin and eosin; Magnification ×400.
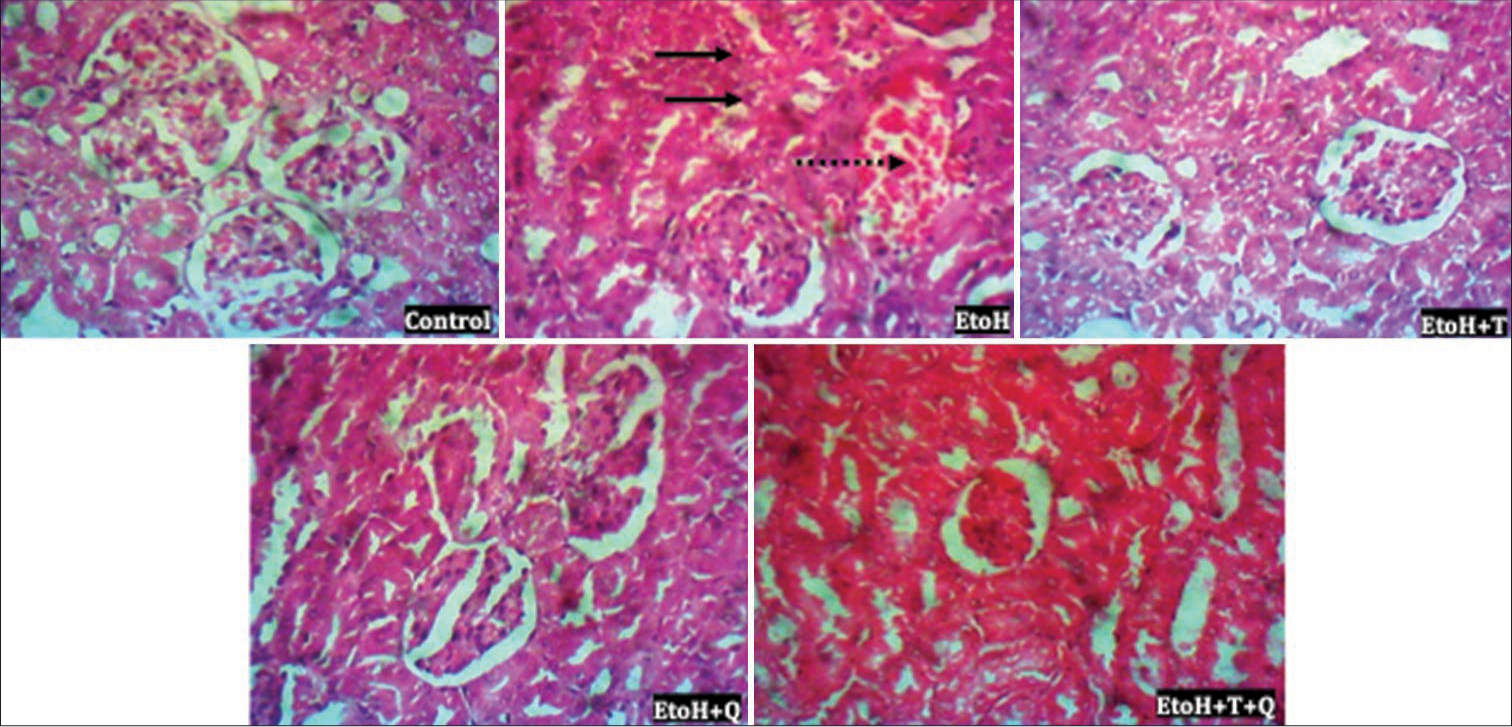
- Photomicrograph of renal tissue showing patchy tubular necrosis and ectasia (thick black arrow) and congestion (broken arrow). Hematoxylin and eosin (H&E); Magnification ×400 Heart; H&E; Magnification ×400.
![The immunohistochemistry of cardiac troponin. (a) Group A (Control), (b) Group B (Ethanol [EtoH] [40%]), (c) Group C (EtoH [40%] + alpha-tocopherol (2.5 mg/kg), (d) Group D (EtoH [40%] + quercetin 50 mg/kg), and (e) Group E (EtoH [40%] + alpha-tocopherol + quercetin 50 mg/kg). Black arrows indicate immunohistochemical expression of cardiac troponin. Slides stained with high definition hematoxylin. (Magnification ×100).](/content/138/2024/4/1/img/AJBPS-4-6-g014.png)
- The immunohistochemistry of cardiac troponin. (a) Group A (Control), (b) Group B (Ethanol [EtoH] [40%]), (c) Group C (EtoH [40%] + alpha-tocopherol (2.5 mg/kg), (d) Group D (EtoH [40%] + quercetin 50 mg/kg), and (e) Group E (EtoH [40%] + alpha-tocopherol + quercetin 50 mg/kg). Black arrows indicate immunohistochemical expression of cardiac troponin. Slides stained with high definition hematoxylin. (Magnification ×100).
![The immunohistochemistry of renal angiotensin converting enzyme. (a) Group A (Control), (b) Group B (Ethanol [EtoH] [40%]), (c) Group C (EtoH [40%] + alpha-tocopherol 2.5 mg/kg), (d) Group D (EtoH [40%] + quercetin 50 mg/kg), and (e) Group E (EtoH [40%] + alpha-tocopherol + quercetin 50 mg/kg). Black arrows indicate immunohistochemical expression of angiotensin converting enzyme. Slides stained with high definition hematoxylin. (Magnification ×100).](/content/138/2024/4/1/img/AJBPS-4-6-g015.png)
- The immunohistochemistry of renal angiotensin converting enzyme. (a) Group A (Control), (b) Group B (Ethanol [EtoH] [40%]), (c) Group C (EtoH [40%] + alpha-tocopherol 2.5 mg/kg), (d) Group D (EtoH [40%] + quercetin 50 mg/kg), and (e) Group E (EtoH [40%] + alpha-tocopherol + quercetin 50 mg/kg). Black arrows indicate immunohistochemical expression of angiotensin converting enzyme. Slides stained with high definition hematoxylin. (Magnification ×100).
DISCUSSION
The oxidation of lipids in the milieu intérieur is modifiable by systemic antioxidative compounds that occur naturally in combinations with overall effects that are usually greater than that of individual agents acting alone and are thus described as synergistic effects.[36] In this study, the modulatory and synergistic roles of two dietary antioxidants, alpha-tocopherol and quercetin, were investigated in EtoH-associated derangements of cardiovascular system. Redox imbalances have been prominently implicated in several multi organ-system pathologies including those of the cardiovascular and renal systems,[37] and alcohol has been reported to mediate some of its toxicities by tilting the redox balance in favor of the pro-oxidants particularly in the cardiorenal tissues. EtoH-induced oxidative stress may exacerbate the participation of reactive oxygen species (ROS) as second messengers in vascular endothelium-dependent function and remodeling of blood vessels; with the dysregulation of these physiological processes having considerable potential of contributing significantly to the development of vascular diseases.[38] When more than the coping capacity of the systemic antioxidants, the prooxidative agents cause significant deleterious effects on mammalian organs and tissues.[39] Therefore, the observation of attenuation of oxidative stress manifested by reduced lipid peroxidation, lowered hydrogen peroxide production, as well as lowered MPO and sulfhydryl thiols by alpha-tocopherol and quercetin suggest a potent activity for the administered dietary antioxidants to reduce oxidative stress, as previously described by various authors.[40,41]
In biological systems, alpha-tocopherol potently attenuates peroxidative damage in organs, with an inverse correlation reported between the level of the antioxidant and physiological derangements in the cardiovascular system.[42] Likewise, quercetin reportedly mitigates drug-instigated cardiomyopathy by positively modulating enzymatic antioxidative agents in rats.[43] In this study, the levels of the analyzed systemic antioxidants GSH, GPx, SOD, and GST are significantly reduced following alcohol exposure. Thus, it can be inferred that alcohol exerted a potent oxidative stress inducing activity by producing large amounts of ROS that required heightened mopping up by the systemic enzymatic and non-enzymatic antioxidants and consequent depletion of the agents for the restoration of physiological oxidant levels in cardiovascular and renal systems. Alcohol-induced oxidative stress is linked to the metabolism of EtoH involving both microsomal and mitochondrial systems, production of ROS and reactive nitrogen species, elevation of MDA, hydroxyethyl radical, and hydroxynonenal protein adducts, depletion of GSH levels, decreased antioxidant activity, modification of all biological structures, and serious malfunction of cells and tissues.[44] Moreover, hydrogen peroxide has been reported to increase the production of endogenous vasoconstrictors in blood vessels, thereby inducing increased arteriolar tone and consequently hypertension.[45] The potentiation of the antioxidant effect of alpha-tocopherol by quercetin and vice versa recorded in this study ratifies the submission of Punithavathi and Prince,[46] who reported that the combination of the two antioxidants is synergistic and potently scavenge-free radicals, with the improvement of systemic antioxidant capacity and maintenance of calcium ion levels. High levels of alcohol in mammalian tissues downregulate endothelial NO synthesis, alter bioavailability of NO, and impair vascular relaxation in rats, thereby predisposing the cardiovascular system to hypertension and other diseases such as renal failure.[47,48] Therefore, the significantly elevated serum NO when rats were gavage with alpha-tocopherol and quercetin compared with rats exposed to the toxic effects of alcohol without antioxidant treatment further corroborates a positive modulatory role for the two antioxidants in alcohol-induced hypertension and associated renal dysfunction.
The assessment of creatinine and urea nitrogen levels has replaced the classically used inulin clearance by the kidneys in the measurement of glomerular filtration rate and accurate determination of the functional status of the kidneys.[49,50] From our results, a significant increase seen in creatinine/urea levels following alcohol exposure without antioxidant treatment may suggest a reduction in renal functional activity and has been reported as a common complication of acute kidney injury.[51] Under physiological conditions, 40–60% of filtered urea in the glomerular filtrate is conserved by reabsorption through the renal tubules, but this becomes significantly altered when the kidneys are not performing optimally.[52] Creatinine, produced from the breakdown of creatine and phosphocreatine, circulates in the blood, and is solely eliminated by the kidneys; thus, constituting renal function marker.[53,54] Therefore, observation of lower urea and creatinine levels in rats treated with alpha-tocopherol and quercetin and their combinations suggest potent protective effects for the two antioxidants especially when used in combination. This assertion is further strengthened by the absence of histopathologic lesions in the renal tissues of rats treated with alpha-tocopherol, quercetin, and their combination. Reports from this study corroborate earlier reports of Zal et al.,[55] who reported induction of lowered renal functional markers following administration of quercetin/Vitamin E cocktail to cyclosporine administered rats. Vitamin E is a chain breaking antioxidant, whose mechanism of action manifests during lipid peroxidation, with the hydroxyl group of the Vitamin E reacting with the peroxyl radical to form the corresponding lipid hydroperoxide and the chromanoxyl radical.[56] Quercetin has also been reported to improve renal function by reducing the levels of creatinine, BUN, and urinary uric acid in a rat model of adenine-induced chronic kidney disease.[57] Likewise, using chromium as the toxicant, quercetin in combination with N-acetylglucosamine exerted a dose-dependent efficacy demonstrated by a significant increase in animal survival, renal excretory function, and normalization of nitrogen metabolism in rats.[58] Moreover, alpha-tocopherol reportedly exerts an antiatherogenic effect by ameliorating oxidative processes, thereby reducing formation of atheromatous plaques, morbidity and mortality in renal failure patients.[59]
Furthermore, immunohistochemical evaluation revealed alcohol-induced increased expressions of cTn I in cardiac tissues of rats compared with those that received the antioxidants either singly or in combination, although with a significantly lowered expression of cTn I in the rats treated with the combination rather than individual treatment. The cTn I is a useful biomarker for the detection of other cardiac pathologies including acute coronary syndromes.[60] Accumulating evidence has indicated a unique role for inflammation in EtoH-induced organ damage, including cardiotoxicity.[61] Similarly, immunohistochemical staining of paraffin embedded renal tissues of rats revealed significant attenuation of ACE expression in alpha-tocopherol and quercetin administered rats compared with the rats exposed to only the toxic effects of alcohol. The ubiquitously distributed ACE stimulate the formation of an endogenous vasoconstrictor – angiotensin II. The observation of heightened expression of ACE in rats exposed to the toxic effects of alcohol without antioxidant treatment points to an intensification of Ang II formation from increased ACE activity with consequent hypertension. The QT interval on surface electrocardiogram represents the sum of depolarization and repolarization process of the ventricles,[62] and its prolongation, has been pathophysiologically linked with decreases in repolarizing outward potassium currents or increases in depolarizing inward sodium or calcium currents.[63] Various factors, including exposures to drugs and chemicals have been reported to prolong QT interval and increase predisposition to arrhythmia.[64] The observation of prolonged QTc in this study corroborate an earlier report of Li et al.[65] who linked heavy alcohol consumption with increased predisposition to prolonged QTc interval in human subjects. Therefore, normalization of the QTc interval in rats treated with alpha tocopherol and quercetin, in this study, strongly suggest potent cardioprotective effects for these antioxidants.
CONCLUSION
This study concludes that the oral administration of alpha-tocopherol and quercetin significantly ameliorated alcohol-mediated changes of primary hemodynamic parameters, electrocardiographic changes, antioxidant defense status, renal functional markers, histopathogic changes, and immunohistochemical expressions of cTn I and ACE.
Ethical approval
The research/study approved by the Institutional Review Board at University of Ibadan, number TOA/20/0036, dated April 5, 2020.
Declaration of patient consent
Patient’s consent not required as there are no patients in this study.
Conflicts of interest
There are no conflicts of interest.
Use of artificial intelligence (AI)-assisted technology for manuscript preparation
The authors confirm that there was no use of artificial intelligence (AI)-assisted technology for assisting in the writing or editing of the manuscript and no images were manipulated using AI.
Financial support and sponsorship
Nil.
References
- Alcohol consumption and cardiovascular disease. A Mendelian randomization study. Circ Genom Precis Med. 2020;13:e002814.
- [CrossRef] [PubMed] [Google Scholar]
- Antioxidant treatment and alcoholism In: Molecular aspects of alcohol and nutrition. Molecular Aspects of Alcohol and Nutrition. Netherlands: Elsevier; 2016. p. :119-31.
- [CrossRef] [Google Scholar]
- Alcohol use among adolescents in India: A systematic review. Glob Ment Health. 2022;9:1-25.
- [CrossRef] [PubMed] [Google Scholar]
- Alcohol use and burden for 195 countries and territories, 1990-2016: A systematic analysis for the Global Burden of Disease Study 2016. Lancet. 2018;392:1015-35.
- [CrossRef] [PubMed] [Google Scholar]
- Metabolic profiling of alcohol consumption in 9778 young adults. Int J Epidemiol. 2016;45:1493-506.
- [CrossRef] [PubMed] [Google Scholar]
- Evidence for a direct harmful effect of alcohol on myocardial health: A large cross-sectional study of consumption patterns and cardiovascular disease risk biomarkers from Northwest Russia, 2015 to 2017. J Am Heart Assoc. 2020;9:e014491.
- [CrossRef] [PubMed] [Google Scholar]
- Consumption of alcohol and blood pressure: Results of the ELSA-Brasil study. PLoS One. 2018;13:0190239.
- [CrossRef] [PubMed] [Google Scholar]
- Alcohol-induced hypertension: Mechanism and prevention. World J Cardiol. 2014;26:245-52.
- [CrossRef] [PubMed] [Google Scholar]
- Hypertension and chronic ethanol consumption: What do we know after a century of study? World J Cardiol. 2014;6:283-94.
- [CrossRef] [PubMed] [Google Scholar]
- Oxidative effects of chronic ethanol consumption on the functions of heart and kidney: Folic acid supplementation. Alcohol Alcohol. 2012;47:404-12.
- [CrossRef] [PubMed] [Google Scholar]
- Oxidative damage to proteins and DNA in rats exposed to cadmium and/or ethanol. Chem Biol Interact. 2009;180:31-8.
- [CrossRef] [PubMed] [Google Scholar]
- Chrysin modulates ethanol metabolism in wistar rats: A promising role against organ toxicities. Alcohol Alcohol. 2011;46:383-92.
- [CrossRef] [PubMed] [Google Scholar]
- Preventing and mitigating alcohol toxicity: A review on protective substances. Beverages. 2018;4:39.
- [CrossRef] [Google Scholar]
- New mechanisms of action of natural antioxidants in health and disease II. Antioxidants. 2021;10:1200.
- [CrossRef] [PubMed] [Google Scholar]
- Ethanol: Striking the cardiovascular system by harming the gut microbiota. Am J Physiol Heart Circ Physiol. 2021;321:275-29.
- [CrossRef] [PubMed] [Google Scholar]
- In vivo effects of vanadium pentoxide and antioxidants (ascorbic acid and alpha-tocopherol) on apoptotic, cytotoxic, and genotoxic damage in peripheral blood of mice. Oxid Med Cell Longev. 2016;2016:6797851.
- [CrossRef] [PubMed] [Google Scholar]
- α-Tocopherol at nanomolar concentration protects cortical neurons against oxidative stress. Int J Mol Sci. 2017;18:216.
- [CrossRef] [PubMed] [Google Scholar]
- Hepatoprotective effects of vitamin E against hexachlorobenzene-induced hepatotoxicity and oxidative stress in rats: Histological, biochimical and antioxidant status changes. Toxicol Mech Methods. 2019;29:18-25.
- [CrossRef] [PubMed] [Google Scholar]
- α-Tocopherol protects the heart, muscles, and testes from lipid peroxidation in growing male rats subjected to physical efforts. Oxid Med Cell Longev. 2019;2019:8431057.
- [CrossRef] [PubMed] [Google Scholar]
- Quercetin exhibits potent antioxidant activity, restores motor and non-motor deficits induced by rotenone toxicity. PLoS One. 2021;16:e0258928.
- [CrossRef] [PubMed] [Google Scholar]
- Effect of crocin, an active saffron constituent, on ethanol toxicity in the rat: Histopathological and biochemical studies. Iran J Basic Med Sci. 2020;23:51-62.
- [Google Scholar]
- α-Tocopherol preserves cardiac function by reducing oxidative stress and inflammation in ischemia/reperfusion injury. Redox Biol. 2019;26:101292.
- [CrossRef] [PubMed] [Google Scholar]
- Quercetin and Vitamin C mitigate cobalt chloride-induced hypertension through reduction in oxidative stress and nuclear factor kappa beta (NF-Kb) expression in experimental rat model. Biol Trace Elem Res. 2017;175:347-59.
- [CrossRef] [PubMed] [Google Scholar]
- Supplementation of antihypertensive drug regimen with vitamin E ameliorates alterations of primary haemodynamic parameters and total antioxidant capacity in ovariectomised rats. J Basic Clin Physiol Pharmacol. 2021;34:33-9.
- [CrossRef] [PubMed] [Google Scholar]
- Sodium fluoride induces hypertension and cardiac complications through generation of reactive oxygen species and activation of nuclear factor kappa beta. Environ Toxicol. 2016;32:1089-101.
- [CrossRef] [PubMed] [Google Scholar]
- Ferrous ion oxidation in the presence of ferric ion indicator xylenol orange for measurement of hydrogen peroxides. Methods Enzymol. 1994;233:182-9.
- [CrossRef] [Google Scholar]
- The role of superoxide anion in the autoxidation of epinephrine and a simple assay for superoxide dismutase. J Biol Chem. 1972;217:3170-5.
- [CrossRef] [PubMed] [Google Scholar]
- Glutathione-S-transferases. The first enzymatic step in mercapturic acid formation. J Biol Chem. 1974;249:7130-9.
- [CrossRef] [PubMed] [Google Scholar]
- Improved method for the determination of blood glutathione. J Lab Clin Med. 1963;61:882-8.
- [Google Scholar]
- A rapid, simple spectrophotometric method for simultaneous detection of nitrate and nitrite, Nitric Oxide. J Biol Chem. 2001;5:62-71.
- [CrossRef] [PubMed] [Google Scholar]
- Measurement of myeloperoxidase in leukocyte-containing tissues. Anal Biochem. 1997;245:93-6.
- [CrossRef] [PubMed] [Google Scholar]
- Carlton's histopathological techniques (4th ed). London: Oxford University Press; 1976.
- [Google Scholar]
- Synergism and antagonism between quercetin and other chain-breaking antioxidants in lipid systems of increasing structural organization. Food Chem. 2007;103:1288-96.
- [CrossRef] [Google Scholar]
- Exercise and oxidative stress: Potential effects of antioxidant dietary strategies in sports. Nutrition. 2015;31:916-22.
- [CrossRef] [PubMed] [Google Scholar]
- Reactive oxygen species in the vasculature: Molecular and cellular mechanisms. Hypertension. 2003;42:1075-81.
- [CrossRef] [PubMed] [Google Scholar]
- Oxidative stress and hypertension: Possibility of hypertension therapy with antioxidants. J Res Med Sci. 2014;19:358-67.
- [Google Scholar]
- Protective effect of selenomethionine on aflatoxin B1-induced oxidative stress in MDCK cells. Biol Trace Elem Res. 2014;157:266-74.
- [CrossRef] [PubMed] [Google Scholar]
- Lifestyle, oxidative stress, and antioxidants: Back and forth in the pathophysiology of chronic diseases. Front Physiol. 2020;11:694.
- [CrossRef] [PubMed] [Google Scholar]
- Protective effect of exercise and alpha tocopherol on atherosclerosis promotion in hypercholesterolemic domestic rabbits. AIP Conf Proc. 2017;1888:020049.
- [CrossRef] [Google Scholar]
- Modulation of Nrf2 by quercetin in doxorubicin-treated rats. Heliyon. 2020;6:e03803.
- [CrossRef] [PubMed] [Google Scholar]
- H2O2 increases production of constrictor prostaglandins in smooth muscle leading to enhanced arteriolar tone in Type 2 diabetic mice. Am J Physiol Heart Circ Physiol. 2007;292:649-56.
- [CrossRef] [PubMed] [Google Scholar]
- The cardioprotective effects of a combination of quercetin and α-tocopherol on isoproterenol-induced myocardial infarcted rats. J Biochem Mol Toxicol. 2011;25:28-40.
- [CrossRef] [PubMed] [Google Scholar]
- Blood vessels as a key mediator for ethanol toxicity: Implication for neuronal damage. Life. 2022;12:1882.
- [CrossRef] [PubMed] [Google Scholar]
- Flow-mediated dilation and cardiovascular risk prediction: A systematic review with meta-analysis. Inter J Cardiol. 2013;168:344-51.
- [CrossRef] [PubMed] [Google Scholar]
- Renal biomarkers of male and female Wistar rats (Rattus norvegicus) undergoing renal ischemia and reperfusion. Acta Cir Bras. 2015;30:277-88.
- [CrossRef] [PubMed] [Google Scholar]
- Methods for assessment of the glomerular filtration rate in laboratory animals. Kidney Dis. 2022;8:381-91.
- [CrossRef] [PubMed] [Google Scholar]
- Acute kidney injury: Definition, pathophysiology and clinical phenotypes. Clin Biochem Rev. 2016;37:85-98.
- [Google Scholar]
- Urea and ammonia metabolism and the control of renal nitrogen excretion. Clin J Am Soc Nephrol. 2015;10:1444-58.
- [CrossRef] [PubMed] [Google Scholar]
- Predictive value of serum myoglobin and creatine phosphokinase for development of acute kidney injury in traumatic rhabdomyolysis. Indian J Crit Care Med. 2017;21:852-6.
- [CrossRef] [PubMed] [Google Scholar]
- Comparison of the effects of vitamin E and/or quercetin in attenuating chronic cyclosporine A-induced nephrotoxicity. Clin Exp Pharmacol Physiol. 2007;34:720-4.
- [CrossRef] [PubMed] [Google Scholar]
- Quercetin and its role in biological functions: An updated review. EXCLI J. 2018;17:856-63.
- [Google Scholar]
- N-acetylglucosamine increases the efficacy of quercetin in the treatment of experimental acute kidney injury. J Pharm Pharmacogn Res. 2019;8:53-63.
- [CrossRef] [Google Scholar]
- Antioxidant supplementation in renal replacement therapy patients: Is there evidence? Oxid Med Cell Longev. 2019;2019:9109473.
- [CrossRef] [PubMed] [Google Scholar]
- Myocardial tissue troponins T and I. An immunohistochemical study in experimental models of myocardial ischemia. Cardiovasc Pathol. 2003;12:65-71.
- [CrossRef] [PubMed] [Google Scholar]
- Selective inhibition of the NLRP3 inflammasome protects against acute ethanol-induced cardiotoxicity in an FBXL2-dependent manner. Acta Biochim Biophys Sin. 2023;55:1972-86.
- [CrossRef] [PubMed] [Google Scholar]
- Glomerular localization and expression of angiotensin-converting enzyme 2 and Angiotensin-converting enzyme: Implications for albuminuria in diabetes. J Am Soc Nephrol. 2006;17:3067-75.
- [CrossRef] [PubMed] [Google Scholar]
- Normal ventricular repolarization and QT interval: Ionic background, modifiers, and measurements. Card Electrophysiol Clin. 2017;9:487-513.
- [CrossRef] [PubMed] [Google Scholar]
- Long-QT syndrome: From genetics to management. Circ Arrhythm Electrophysiol. 2012;5:868-77.
- [CrossRef] [PubMed] [Google Scholar]
- QTc prolongation in poison center exposures to crediblemeds list of substances with “known risk of torsades de pointes”. Cardiovasc Toxicol. 2022;22:866-77.
- [CrossRef] [PubMed] [Google Scholar]
- Relation of heavy alcohol consumption to QTc interval prolongation. Am J Cardiol. 2016;118:1201-6.
- [CrossRef] [PubMed] [Google Scholar]